The Revalue Chain
This map illustrates some of potential complementaries and alternatives to 'production-as-usual,' in relation to the existing ‘Linear Value Chain.' It was initially developed to clearly communicate what remanufacturing is, and how it fits within this eco-system. Therefore, there is some bias towards remanufacturing in the main text below, and in the 'Design for Revalue' processes.
As the map is based on the Linear Value Chain, it maybe advisable to watch a short introduction to what that is first, before going into further detail on this page.

Initially developed as part of a first regional remanufacturing initiative, that was provoked in the Basque Country, by Acede (an industrial cluster), H-Enea Living-Lab and the Provincial Government of Gipuzkoa, entitled ‘Reman Gipuzkoa.’ The map and the presentation was developed by Agence designContext, and was facilitated by Régis Dando of remanufacturing.fr; with the stakeholder development being managed and dynamised by H-Enea Living Lab. The first meeting, in 2015, was entitled ‘Reman 101,’ which had the dual objective of creating an initial dialogue between key ‘soft infrastructure’ actors (political, economical, environmental, industrial clusters, innovation, media, university…), those industries and social groups already involved in revalue activities in the region (Emmaus, Zero Waste Gipuzkoa, Motorlan, ReBattery…), mixed with an intensive overview of the revalue chain - to build a shared level of understanding and language between all involved.
Since this initial work, projects have been developed, and more are currently in development, and the understanding of the potential of remanufacturing has spread across the Basque region. Remanufacturing is now one of the key governmental circular economy strategies in the region.
Below is a short list of some of the actors already active around the world, which can help in understanding of the map, and the typology. Each icon in the map above is listed below to the left, with examples to the right: Capital Goods Industries: (Industrial Equipment): Retec Machines (FR).
Service Industries: Deutsche Post DHL, Vespertec, Recover-e(NL), UPS with iQor Aftermarket Solutions, Round Trip Logistics.
Component Industries: Valeo (FR), SKF (SW), JEGS (US).
Turnkey Suppliers: (sub-contracted revalue suppliers): Flextronics, Fossil Monswiller Site, (Tiama) (DE).
Subsidiaries: Renault subsidiary in Choisy-le-Roi (FR), Ricoh UK (UK).
Lead Production Firms: Caterpillar Inc., Xerox Inc., Rolls Royce, John Deere, Neopost (FR), Interface Flor.
Retailers and Resellers: Fnac (FR), Stormfront (UK), mResell(UK), Apple Store, Cash Converters, Cash Express.
After-Sales-Services: Candy (UK), Alltrucks (DE).
D.I.Y Services: iFixit, London Bike Kitchen (UK), Repair Café.
Core Brokers: European Recycling Platform, Eco-systèmes(FR), Ecolec (SP), Autoenterprises (UK), Swico Recycling (CH).
Maintenance: Lufthansa Technik (DE), AFI KLM E&M.
Refurbishment: Emmaus, Envie (FR), Tiama.
Recondition: EnvironCom (UK), Motorlan (SP).
Remanufacture: Armor (FR), Robotif GmbH (D), Lizarte (SP), SRC, Weichai Reman Company, Flight Systems European Group, BU Drive (DE).
Recycling: Veolia (FR), Clamens (FR), Misapor (CH), Earth Stone International, Preserve.
Manufacturing & AFFF Infrastructure: Catapult: High Value Manufacturing (UK).
Principles/Standards/Guidelines: British Standard Institute(UK).
Development Policy: The All-Party Parliamentary Sustainable Resource Group UK.
Parks & Zones: Kalunborg Symbiosis, John Deere Reman – Strafford Industrial Park.
Financial Institutions: Green Bank (UK).
R&D Labs: GSCOP (FR), R.I.T Centre for Remanufacturing(US), Advanced Remanufacturing and Technology Centre (SG), Fraunhofer.
Higher Education: University of Strathclyde (Scotland), WASEDA University (JP), Nanyang Technological University(Singapore), Fraunhofer IPA & University of Bayreuth (DE). TUBerlin (DE).
Knowledge Networks: Ellen MacArthur Foundation (UK), ERN, Duxes Reman Industry Focus, The Knowledge Transfer Network(KTN) and _connect (UK), High Speed Sustainable Manufacturing Institute, University of Cambridge Institute (UK), WRAP (UK), APRA.
Action Groups: Zero-Waste Scotland, The All-Party Parliamentary Sustainable Resource Group UK, Innovate UK: Technology Strategy Board, Scottish Institute for Remanufacture, and H-Enea Living Lab (SP).
Altieri, Miguel A. Nicholis, Clara I. (2005 – English version) ‘Agroecology and the Search for a Truly Sustainable Agriculture.’ 1st Edition. 9 Basic Textbooks for Environmental Training. United Nations Environment Programme,, Mexico D.F.
American Community Gardening Association. ‘What is a community garden?’ Retrieved 27/11/2012, from American Community Gardening Association: http://www.communitygarden.org/ learn/
APSRG (The All-Party Parliamentary Sustainable Resource Group) (March 2014), ‘Remanufacturing: Towards a Resource Efficient Economy.’ UK.
APSRG (The All-Party Parliamentary Sustainable Resource Group) (December 2014), ‘Triple Win: The Economic, Social and Environmental Case for Remanufacturing’. UK.
Capra, Fritjof and Luisi, Pier Luigi. (2014) ‘The systems view of life: a unifying vision.’ University Printing House, Cambridge CB2 8BS, United Kingdom.
Chatham House, Lee, Bernice et al., (December, 2012) ‘Resource Futures.’ A Chatham House Report. London: The Royal Institute of International Affairs. UK.
Chiodo, Dr. Joseph. (January 2005) ‘Design for Disassembly Guidelines, Active Disassembly Research’. Retrieved 26/06/2015, from www.activedisassembly.com
Creasy, R. (2009) ‘Edible Landscaping Basics.’ Retrieved 17/11/2015, from Rosalind Creasy – Edible Landscaping: http://www.rosalindcreasy.com/edible-landscaping-basics/
EcoDesign Resource Society (EDRS) (2013) ‘The Urban Farming Guidebook: Planning for the Business of Growing Food in BC’s Towns & Cities.’
EMF (Ellen MacArthur Foundation), (2012) ‘Towards the circular Economy: Economic and business rationale for an accelerated transition.’ UK.
EMF (Ellen MacArthur Foundation) & D&T (The Design and Technology Association), (2012/V1.1) ‘SYSTEM RESET: Design and Technology for a circular economy: Teachers’ Guide.’ UK.
EMF (Ellen MacArthur Foundation), (2015) ‘Delivering the Circular Economy: A Toolkit for Policymakers.’ UK. 1
EEF The manufacturers’ organisation(July 2014) ’Materials for Manufacturing: Safeguarding Supply’. UK.
EEF in partnership with Aldermore(2014, 2013, 2012) ‘Executive Survey 2014, 2013, 2012: Manufactures’ views on the economy and their own prospects in 2014.’
European Commission (2011) Roadmap to a Resource Efficient Europe, SEC (2011) 1067 final; COM (2011) 571 final. Brussels.
Gallo, Mosè. Romano, Elpidio, and Carmela Santillo Liberatina (2012) ‘A Perspective on Remanufacturing Business: Issues and Opportunities’. licensee InTech.
Geng, Y., et al. ‘Measuring China’s Circular Economy’. Science, March 2013.
Gray, Casper. and Charter, Martin(2007) ‘Remanufacturing and Product Design: Designing for the 7th Generation.’ The Centre for Sustainable Design. University College for the Creative Arts, Farnham, UK
Giuntini, Ron and Gaudette, Kevin(2003). ‘Remanufacturing: The next great opportunity for boosting US productivity.’
Gliessman, S.R. (1998) ‘Agroecology: ecological processes in sustainable agriculture.’ Ann Arbor Press, Michigan.
ICMM, International Council on Mining & Metals. (October, 2012) ‘In Brief: Trends in the mining and metals industry: Mining’s contribution to sustainable development.’ ICMM 35/38 Portman Square, London.
Ijomah, Winfred. (2002) “A Model-Based Definition of the Generic Remanufacturing Business Process.’ doctoral thesis completed at University of Plymouth Enterprise.
Ijomah, Winfred L. et al. (2007) ‘Development of design for remanufacturing guidelines to support sustainable manufacturing’. Robotics and Computer-Integrated Manufacturing 23 712–719. University of Bath, Bath, UK.
Ijomah, Winifred L. (2009) ‘Addressing decision making for remanufacturing operations and design-for-remanufacture’, International Journal of Sustainable Engineering, 2:2, 91-102, DOI: 10.1080/19397030902953080
ING Economics Department (May, 2015) ‘Rethinking finance in a circular economy.’
Klein, Naomi. (2014) ‘This Changes Everything: Capitalism Vs. the Climate. Penguin Books Ltd, London, England.
Lacy. P., Keeble, J., McNamara, R., Rutqvist, J., Eckerle, K., Haglund, T., Buddemeier, P., Cui, M., Sharma, A., Cooper, A., Senior, T. and Pettersson, C. (2014). ‘Circular Advantage – Innovative Business Models and Technologies to Create Value in a World without Limits to Growth.’ Accenture
Lovins, Amory. (2009) ‘Reinventing Fire.’ RMI Solutions Journal, Fall.
Millennium Ecosystem Assessment. (2005) ‘Ecosystems and Human Well-being: Synthesis.’ Island Press, Washington, DC. World Resources Institute.
Orwell, George. (1937). ‘The Road to Wigan Pier.’ Retrieved February 17, 2016 from The Complete Works of George Orwell: http://www.george-orwell.org/The_Road_to_Wigan_Pier/index.html
Parker, D. (2007) An introduction to remanufacturing. Oakdene Hollins. Available at http://www.remanufacturing.org.uk/pdf/reman_primer.pdf
Pauli, Gunter. (1998) ‘Upsizing: The Road to Zero Emissions, More Jobs, More Income, and no Pollution.’ Green leaf Publishing. UK.
Pauli, Gunter. (2010) ‘ZEN and the Art of Blue: How to connect the quality of your life to the Blue Planet Earth.’ Convergent Publishing UG (haftungsbechränkt). Berlin, Germany.
Pauli, Gunter. (2015) ‘Case 105 Clustering Biofuels and Heavy Industry.’ Pauli, Blue Economy.
Pretty, J.N. (1994) ‘Regenerating Agriculture.’ Earthscan Publications Ltd., London.
RREUSE (September, 2015) ‘Improving product reparability: Policy options at EU level.’ The Reuse and Recycling EU Social Enterprise network.
Schinzing, Russ (07 July 2010) ‘Cores-Cores-Cores’. Available at http://e-reman.com/blog/cores-cores-cores/ Viewed the 02/05/2015.
SERI Working Papers (No. 9, July 2009) ’A comprehensive set of resource use indicators from the micro to the macro level.’ Stefan Giljum et al., SERI Sustainable Research Institute.
Stahel, Walter R. (2014) ‘The Business Angle of a Circular Economy: Higher Competitiveness, Higher Resource and Security and Material Efficiency.’ in ‘A New Dynamic Effective Business in a Circular Economy.’ Edited by the Ellen MacArthur Foundation. Ellen MacArthur Foundation Publishing. UK.
Stahel, Walter R. and Reday, G. (1976) ‘The potential for substituting manpower for energy, report to the Commission of the European Communities, Brussels.’
Stahel, Walter R. and Reday, G. (1981) ‘Jobs for Tomorrow, the potential for substituting manpower for energy, Vantage Press, New York, N.Y.
Steinhilper, Rolf. (2000) ‘Remanufacturing: The Ultimate Form of Recycling.’…
Sundin Erik. (2004) ‘Product and Process Design for Successful Remanufacturing’. Department of Mechanical Engineering. Linköping, Sweden: Linköping University.
Sundin et al. (2013) ‘Reverse logistics challenges in remanufacturing of automotive mechatronic devices.’ Journal of Remanufacture. Springer.
The Organic Stream (Dec, 2015) ‘#40 The Anaerobic Digestion Update: Using Operational Efficiency & Better Policy to Solve Current Challenges.’ The Organic Stream, Malmö Sweden.
USITC (United States International Trade Commission) (October 2012) ‘Remanufactured Goods: An Overview of the U.S. and Global Industries, Markets, and Trade.’ USITC Publication 4356, Investigation No. 332-525. Washington, DC 20436.
Zavodska, Anita. Uhuo Jerry, E. Benesova Libuse. ‘Contrasting Developed And Developing Countries.’ CHEMICAL ENGINEERING AND CHEMICAL PROCESS TECHNOLOGY – Resource Recycling And Reuse. Encyclopaedia of Life Support Systems (EOLSS)



From an ecological perspective, the predominant linear ways that we collectively extract, consume or retain, and discard materials is no longer viable at the current scales and rates, due to the negative effects that these activities have on Earth’s biogeochemical (i.e., carbon, nitrogen, oxygen, and water) cycles, and ecological systems (all life). These activities also create feedback loops, that reduce Earth’s abilities to continue to produce these materials (at current scales and rates), and reabsorb them safely after we discard them. Our production systems (agricultural and industrial) are a key part of how we provision for many of our basic needs, including food, fresh water, health, quality-of-life, shelter, clothing, wealth creation and distribution (and access), and jobs (types and availability) for instance. The Revalue Chain groups and highlights many of the strategies that have been developed, around the world, that both attempt and prove to go beyond the linear model, creating new and improved ways for production systems to not only work within natural systems, but also, potentially regenerate natural systems by design. Compared to the Linear Value Chain, the Revalue Chain looks far more complicated – and that is because it is… The linear model is a (relatively) simple process – hence it’s dominance, and success. ‘Circular’ value chains/networks, are inherently far more complicated by the very nature, simply as there are many more elements and competencies in the process that need to be considered. This links to the reality that everything is connected, and so all parts have an influence on other parts of the system, requiring greater quantities and qualities of relationships between elements, and understandings between them. ‘End-of-pipe’ vs. ‘Start-of-pipe’ This thought came out of a discussion with a friend: Many of the concepts shown in the Revalue Chain (except some, such as upcycling and design for revalue) can be seen as ‘end-of-pipe‘ (solutions developed for wasted/used material that have already been produced); or ‘start-of-pipe‘ (solutions developed for the extraction/production/transformation of materials before use). Both are relevant: one can go to the cause/source of the problem (the ‘start-of-pipe’) – and stop it, so that the effect (the ‘end-of-pipe’) is reduced or stopped. However, this can sometimes be a difficult and slow process that can also be too large for direct action – and so nothing is actually done. However, ‘end-of-pipe’ solutions can often be easier to tackle, and if managed well, these projects can also build awareness and solutions that tackle the cause of the problem.
Below is a short overview of how the Revalue Chain could effect the factors of production (Entrepreneurs, Labour, Capital Goods, and Land Area). Entrepreneurs: Entrepreneurs are those willing to bear risk, innovate and develop strategies, manage and lead an activity (economic or non-economic). In the continued transition from Linear Value Chains towards Revalue Chains, entrepreneurship is almost a prerequisite. It is a risk to change the status-quo, and there are a fair number of hurdles to be overcome, with plenty of unproven territories ahead. Revalue activities are systemic. The ‘Entrepreneurs’ working in Revalue will have to be able to work and collaborate across industries and sectors: the inward focus within the company, is a priority that will have to be shared with a outward focus to the ecosystem around the company. Specialised Labour: Firms hire people either as a salary or wage worker, in exchange for their labour. Specialised labour is a slightly more defined description of ‘labour’, as today, many jobs require some form of basic training (which can also be referred to as ‘human capital’). The Revalue Chain is seen as a way to create new jobs (and new types of jobs). According to Steinhilper (2000), remanufacturing for instance, can be a strategy to ‘re-shore’ skilled jobs, in manufacturing, in developed countries. The reason for this potential ‘reshoring’ of jobs (again, using remanufacturing as an example) is explained by Walter Stahel & EMF (2013): “Compared to the traditional manufacturing process, the labour input of the circular economy is higher as (a) its economies of scale are limited in geographic and volume terms, and (b) remanufacturing comprises additional steps of dismantling, cleaning and quality control, which are absent in manufacturing.” Also, with the example of remanufacture, it is also harder to mechanise/automate the processes, compared with batch production of new products. The variety, and skills required, means that skilled hand labour (low and high skills) is still very important, and so it necessitates human intelligence and experience. According to Gray et al (2007), this can lead to creating jobs that have high job satisfaction. However, certain parts of the process need skilled and highly trained personnel, i.e., for ‘Reworking, Replacing or Upgrading’ components in the ‘Remanufacturing’ reverse-engineering process; or in ‘RE-marketing’ and the application of new ‘Business Models,’ and this put’s pressure on the supply of adequately trained labour for some of these revalue activities. As APSRG (Dec, 2007) highlights in the remanufacturing sector, the availability of skilled staff has been cited as one of the main barriers for remanufacturing in the US, and will probably be the same situation in other countries. Thinking in loops, requires innovators to change quite radically their approach to designing new products, and also for those innovators shifting their Business Model (to providing a service instead of selling a product for example), can also be a huge mental change for marketing and sales departments: “On the customer-facing side, sales and marketing will have to complement their chief focus on generating demand and fulfilling customer requirements with generating greater revenues from the use of products and services instead of the purchase of them. They also need to develop new ways to engage and incentivize customers to use and dispose of their products properly, especially if adopting service-based models where customers no longer have direct ownership of products and, as a result, less incentive to take proper care of them.” (Lacy, P., 2014) Capital Good Industries & Capital Goods (Means of Production): Many revalue activities may need access to new forms of Capital Goods (new cleaning or additive manufacturing technologies for example). This maybe an expense that puts revalue out of reach of some smaller SMEs. See the section on ‘Soft Infrastructure’ and within that ’Manufacturing & AFFF Infrastructure,’ for an example strategy from the UK, that aims to bridge some of these issues. Some OEM Capital Good Manufactures, and non-OEM remanufactures (i.e., Robotif GmbH) are already involved in revalue activities in this the capital goods sector, in that they maybe renting instead of selling the products to manufacturers, and/or have developed facilities to reverse-engineer end-of-cycle Capital Goods. Land Area: Land Area can also be classed as a raw material when making life-cycle-assessments (LCAs), although in this map it is classed as a ‘Factor of Production’ as it is not strictly consumed within the production of the final product, and embedded in the final product. We do extract land (earth), minerals etc., during the production process, but this is then classed as raw materials, and not land area: a hectare of land remains a hectare of land (or sea) before or after it is used for production. Land area is not infinite, and, according to SERI (July 2009), it is one of the most restrictive categories of resources we have. Humanity has been changing the use of the land in many ways, even when we were foragers. Since agriculture began, this has increasingly accelerated: “More land was converted to cropland in the 30 years after 1950 than in the 150 years between 1700 and 1850. Cultivated systems (areas where at least 30% of the landscape is in croplands, shifting cultivation, confined livestock production, or freshwater aquaculture) now cover one quarter of Earth’s terrestrial surface.” (Millennium Ecosystem Assessment, 2005) Industry and Infrastructure have also changed the use of land, particularly through covering it, called ‘Sealing.’ Each year, SERI (July, 2009), explains that fertile land is lost to the constriction of building and infrastructure. Human activities can also drive the degradation of remaining fertile land, and therefore, the quality of the resources that can be provided. According to the Millennium Ecosystem Assessment (2005), fisheries and fresh water is already well beyond levels that can be sustained at current demands, much less future ones. And so, the challenge to all revalue activities, is not only stop this trend, but actually reverse it. This will require a better collective knowledge of how natural systems work and change over time, and integrating production into nature systems.
All sectors of industry can effectively enter into the revalue approach. However, there can be preferable strategies, depending on the product (and the market). For example, it can be preferable that durable goods (such as cars or photo-copiers) go through product life extension systems (i.e., remanufacture, recondition, refurbish, and maintain), rather than recycling systems; and biological material goods (such as cotton or wool textiles), and FMC goods (fast moving consumables), can preferably go through recycling systems (down-cycling, functional recycling, and upcycling). Below is a list of some of the industrial sectors that can potentially go through all of product life extension strategies: Machinery & Equipment; Office Machinery & Computers; Electrical Machinery & Apparatus; Radio, Television, & Communication Equipment & Apparatus; Medical, Precision & Optical Instruments; Watches & Clocks; Motor Vehicles & Semi-trailers; Other Motor Vehicles, Trailers, & Semi-trailers; Other Transport Equipment; and Furniture & Other Manufactured Goods.
Similar to the list of industrial sectors mentioned in the section above, all products can go through revalue strategies. However, durable goods, and biological material goods and FMCs (Fast moving consumables), have different ‘preferable’ (economical, social and environmental) possibilities. This is a list (according to Gray et al, 2007), which defines the key criteria that durable products have to have, to make them suitable for product life extension: This criteria is also synthesised by Steinhilper (2000) in a different way: Here is a short list, according to Gray et al., (2007) of some of the main types of durable products that match the criteria and currently go through a form of production life extension (there are a few exceptions, according to Giuntini et al (2003) such as ink-cartridges, which are non-durable goods, but can also have the life extended):


There are a variety of different scenarios for Reuse:
Reuse by the Same Owner: This is in fact, potentially, the revalue loop that requires the lowest level of raw material consumption and energy, to extend the life of a product. When consumers, reuse a product within their own household/family for the same function – i.e., the original owner has a bike that has been outgrown by one child, that can be handed-down to a younger child.
Reuse by a Different Owner: If the product is not wanted/needed by the original owner and their immediate household, then they have the option to resell it (or give it away).
Re-Sellers (intermediates): Here, the original owner can take the used good to a reseller, where they pay the original owner a price for the product, and resell it at a higher price. To create a sufficient profit between buying and (re)selling, re-sellers often make additional works, such as those detailed in the section below in ‘Product Life Extension.
There are a mix of OEMs re-selling their own products (i.e., Apple Store), specialist re-sellers, re-selling a specific range of products (i.e., Stormfront, mResell), or those re-selling almost any product (i.e., Cash Converters, Cash Express). Emmaus is also an example of a company that ‘Recaptures’ and ‘Reverse-Engineers’ end-of-life products, and has outlets where they can ‘Resell’ the revalue products to customers directly.
Re-Seller Platforms: This includes those internet platforms that put in relation those who have something to sell, with those that are looking to buy (a two sided market). Examples of product re-seller platforms are Ebay, Price Minister, Vivastreet, Leboncoin – or even Freecycle, where the goods are given for free.
The Second-Hand-Market (in general): The second-hand-market is a often a market based on asymmetric information. In many ways, revalue products (in the best cases) are the opposite: revalue manufacturers are often communicating (RE-marketing) as much information as possible about the quality of their revalue products – confidence in quality is a key part of relationship with the customer – who, unlike the second-hand-market, can often be repeat customers (so clearly revalue products need to perform…).
USITC (2012), highlights some more reasons why some consumers may not be attracted to revalue products:
i) The price of a remanufactured product and a new product are too close to justify the effort/potential perceived quality issues.
ii) Products, such as consumer electronics, are changing constantly. Older technology can become less desirable, and/or quickly obsolete.
iii) Linked to the obsolescence of technology highlighted above, the “form factor” (i.e., configuration, interface, size, shape) can also age, and become less desirable.
The known (and in some cases justified) stigma with second-hand-goods, is also another reason why many resellers, add additional services (such as 1 year warranty), or make additional product life extensions (such as new batteries in second-hand-phones), in a way to reduce this potential scepticism of product quality and durability.
There are a number of different scenarios for renting:
Service Providers: There are also many retailers, specialised or generalists who sell new products, and also rent new and/or refurbished goods. See R&M Rentals in the U.K. for example, or Lokeo in France.
These service providers can lease, or rent products. The subtle difference is mostly in the timing, for example; if someone leases a car, they may have it for 1 year for example (and nobody else has access to it), with a contract; however a person who rents a car, may only rent it for a day, and in this case the car is usually in use most of this time; whereas in a lease, the car may be idle most of the time, like an owned car.
And so with the addition of service providers, in the revalue chain ‘Customers‘ evolves into three interconnected groups:
A) Consumers: In this case, consumers are still (currently) the most common form of customer, who gain access to the functionality of a good, simply by buying it. The ownership of the good is transferred from a seller to a customer (an individual or another company), and the customer can do what they want (within the law…) with the product.
B) Users: Users, in this map, pay (unless there is another form of exchange, which will be discussed below) for access to a good and/or labour time for a determined period of time, but they never own the good. It can include renting, or leasing, for example, with some form of contract stipulating the terms of use. Note that the term ‘user’ has other meanings in other models (i.e., Business Model Canvas denotes ‘users,’ as mostly those customers that use a product or service for free – particularly in a ‘freemium’ business model).
C) Collaborative Consumption: This third group is an umbrella term developed by Rachel Botsman and her ‘Collaborative Consumption’ network; and it describes a relationship between consumers and users, whereby consumers (the owner of goods) rent access to these goods for limited periods of time to users. And so, it is possible for consumers to make repeat money (which becomes a form of income) from their own products, without selling them.
This is still an evolving field increased by the internet, and there are many different definitions, and types of activities (and theories) in this area; however this group is placed within ‘Customers’ in this map, as mentioned above, this group either owns the asset (so is first a customer) and then shares access to that asset for monetary, or non-monetary benefits (Sharing Economy), or this group acts as a connector between those who have an asset, and those that want access to that asset (Peer Economy).
There are three main types of relationships currently identified:
Business-to-Consumer (B2C): Aided by the internet, these companies actually own the assets, and facilitate through various services, the temporary access to these assets to their users. Zipcar, or Netflix for example use a membership system to allow access to different cars (Zipcar) or films (Netflix) normally on a 24h-7day-per-week basis. Zipcar, in particular needs economies of scale – i.e., it’s current model only works in cities, where there are enough users to support the large investment in cars, parking, and other elements required by the company to provide its’ service.
Peer-to-Peer (P2P): Aided by internet based companies who act as an enabler between a two sided market – person to person; that-is-to-say, the Peer-to-Peer Platforms connect those people that have excess capacity – a spare bedroom for example – with someone looking to rent a bedroom for example, and the platform takes an added fee-per-transaction. As a market operator, they focus on developing ‘trust’ and reducing risk between both sides (sometimes some forms of insurance coverage, and history of both user and ‘rentier’ experiences for example). Examples include companies such as Airbnb, Inc., BlaBlaCar.com, Uber, Inc. and Lyft, Inc. for example.
Some of these Peer-to-Peer Platforms create new markets through innovations in finding ‘gaps in the law.’ For example, there are laws around the traditional taxi delivery method: when a potential ‘User’ raises their arm to attract a taxi (hail), this frames the following transaction within a particular set of rules and laws. When however, a technology makes it possible for a potential customer to attract a taxi via a mobile app (e-hail), the frame of rules and laws are arguably not the same: and here is the new ‘legal’ space to create a new two sided market.
Peer-to-Peer can create problems for the volume (economies-of-scale) business model of traditional manufacturers:
“The Sharing Platforms business model promotes a platform for collaboration among product users, either individuals or organizations. These facilitate the sharing of overcapacity or underutilization, increasing productivity and user value creation. This model, which helps maximize utilization, could benefit companies whose products and assets have a low utilization or ownership rate. However, today it’s most commonly found among companies specializing in increasing the utilization rate of products without doing any manufacturing themselves, putting considerable stress on traditional manufacturers.” (Lacy, P., 2014)
Business-to-Business: This is where businesses can monetise (rent) their idling/excess capacity to other businesses. For example, aided by the LiquidSpace platform, companies that have empty office space can rent that space on an hourly or monthly basis to companies needing some extra space on a temporary basis.
Here are a few of the possible scenarios for Repair within the Revalue Chain:
A) After-Sales-Service Repair: When the product is still under warranty, this can be a department within the OEM, or Original Retailer, or a Private Repair firm sub-contracted by the previous two entities. If the end-of-cycle product is outside of its’ product warranty, a Private Repair Firm can also work with the customer directly.
If the product has been designed for repair, and the business model has already been put in place, then it is possible that the After-Sales-Services is connected via the internet. Here, the After-Sales-Service is connected to the product, at the products location, either by hard (ethernet cable) or wireless connection. Fault diagnose and even repair (if its software) can be done at a distance, improving both the efficiency of a site visit (the engineer goes knowing better the problem before hand), and improving the over-the-phone diagnosis and repair (i.e., the service team can see in real-time, the results of the customers interventions, due to their instructions). Communication companies like Free in France integrate this into their products and ‘Business Model’ for example.
Large international logistics companies are increasingly becoming part of the After-Sales-Service repair networks – particularly when repair work is sub-contracted by an OEM, and particularly when products are under warranty, but not exclusively, as the example below highlights:
“Logistics service providers are increasingly looking at reverse logistics not only as an opportunity to fill backhaul loads but as an attractive stand-alone business. DHL, for instance, established beverage distribution platforms in the U.K. that include the distribution, refilling, repair and collection of vending machines.” (EMF, 2012) (Bold text added)
B) D.I.Y Services Repair: More recently, there is another group of actors in this space, which is a mix between ‘Customer D.I.Y Repair’ and ‘After-Sales-Services’, which includes new types of ‘D.I.Y Services’ (shown to the left side of the column in the map). This can be free, or for a fee (one-off, or subscription for example), which gives access to a dedicated fixing space (with furniture and tools), where the customer can fix the product either on their own, or get help by other members or specialist experts that may work in the space. An example of one of these is the London Bike Kitchen.
D) Customer D.I.Y Repair: Here, the product is maintained by the original customer (or someone they know, and usually for free). The level of technical experience required to do this, is strongly linked to the quality of, and accessibility to, the necessary information from the OEM or Original Retailer, or from information platforms dedicated to sharing maintenance information, such as iFixit (many professionals also use these types of platforms). This, then directly relates to what extent the product has been designed to be maintainable (See the section on ‘Design For Multiple Cycles’) – such as easy fault detection, or easy access to remove and replace the faulty parts for example. If products are out of warranty, then this or disposal are likely options for consumers, if they don’t want to pay, or can’t afford or warrant the expense for a repair professional, as a new product can often be cheaper…
Fab Labs: are also linked to the D.I.Y Services options available, particularly for those skilled customers, who want to make replacement parts or even upgrade them, themselves. Fab Labs are also part of the ‘Manufacturing & AFFF Infrastructure,’ as they provide the public and private companies access to technologies for making (not quite yet manufacturing) components and final goods.
This is when an original owner, or secondary owner, or an intermediate buyer and seller changes the original use of the product, and turns it into something else. An example of an original owner doing this, is a small home-scale farmer, re-using an old bath, and using it as a small duck bath or worm composting bin (permaculture example). An example of an intermediate buyer and seller, is an artist or artisan buying (or salvaging) waste materials to make furniture or sculptures, or old signs to make hand-bags. Pottias for example, in France, use old car seat belts to make high quality hand bags.
This section looks at product life extension strategies that can and are made in the market (by retailers and customers) and by the market, after the product has already been sold by the original manufacturer to the original distributor or to the original customer.


For more detailed information, take a look at a paper by Electronics Remanufacturing Company (ERC), which can be found here, and a paper by Erik Sundin et al., which can be found here. Firstly, it is useful to understand what a ‘Core’ is (particularly for ‘reindustry’ processes), and so, the following definitions are used: “ ‘Core’ is the term commonly used to describe the component or product that will be retained through the remanufacturing process. ‘Hulk’ is a synonym for ‘core’, used notably by Xerox.” (Gray et al, 2007) “…a core… a used part of any kind. Most of the time this part is not referred to as a core until it has been separated from its original-use application. …The same terminology is used for a part that has only been in an application for 1 hour and the exact same part that has been in an application for 5+ years.” (Schinzing, July 2010) So, now that the ‘Core’ terminology is clearer, here is a breakdown of the different types of ‘Waste Management Groups’ :- those groups that either manage the flow of, and /or the access to, these cores: Municipality Waste Sites: These are publicly management sites, that collect and pre-sort different waste streams. This includes different sites that may specialise in different types of waste, and the different collection and distribution sites used to move waste around. Salvage Yards: This includes private centres, that are effectively also brokerage services (see below), that buy and sell scrap – mostly metals; such as iron, steel, stainless steel, brass, copper, aluminium, zinc, nickel, and lead. Retailer Collection Sites: If a customer wants to dispose of an end-of-cycle product, one option available to them, maybe within the French Ecotax scheme. Here, some WEEE retailers can be obliged to take-back the end-of-cycle product, during delivery of the new product at the customers home. The end-of-cycle product is then taken back to the retailers site, and stocked, ready for collection by the various companies further along the revalue chain. Peddlers: Are, principally individuals that enter into scrap/salvage yards and take things off old products. With simple tools, such as shopping carts, wire cutters, and wrenches, they search for parts, that they then can sell directly to core brokers (see Schinzing, July 2010). This can be both legal, and illegal (black market) work… Professional Core Brokers: Core Brokers, also known as Core Suppliers, are professionals (individuals or groups) who work directly with scarp/salvage yards, with disassembly of cars being there core business. The larger of these brokers often publish catalogs, which then remanufacturers can order used units (see Steinhilper, 2000). The main goal of a Core Broker is to recover parts/assemblies of financial value, and to be able to have an inventory (a stock), that then makes it possible for them to supply the remanufacturing industry with the types/qualities/quantities when they need them. The Core Broker can also take assemblies, such as car engines, apart, to then have further subassemblies, such as gearboxes, starters, generators, pumps etc to have a wider range of units to offer (see Steinhilper, 2000). A Core Broker, can also be an intermediate seller, who buys cores and then disassembles them prior to reselling the subassemblies. Some, can add additional services, such as cleaning, and inspection – though rarely any form of reparation (this is the role of the ‘reindustry’ groups). And in this way some of these intermediate seller core brokers, start to perform like an inventory service: managing the stocks of component parts in warehouses, that can be imported or exported when and where they are needed. Additional services can include logistics provision also (see UTISC, 2012). Core Brokers, can work with peddlers, in sourcing components from scrap yards, and OEM/OES surplus. As experience across the revalue chain increases, remanufacturers have often started to be more selective on the type of components they want or expect. This has put pressure on core brokers to be able to both predict which components will be demanded in the future, and if they can manage this in some way, they are able to charge higher prices for higher demand components. And so, core brokers are able to add value: the remanufacturers send core brokers a bid list for a number of parts, and the core broker provides a quotation, prior to a potential order (Schinzing, July 2010). Examples of these companies range from public waste disposal sites, entrepreneurial individuals like John Manners in the UK; to larger, professional companies such as Autoenterprises, also in the UK. An example of an internet platform, with its’ routes in Industrial Ecology, positioning itself between those that have ‘waste’ that they would like to dispose of, and those that are looking for such ‘waste’ as a low cost input for their business is the U.K.’s National Industrial Symbiosis Programme (NISP). The platform also develops face-to-face group events to speed up awareness and opportunities for collaborating, and NISP can work as a brokerage service between two businesses wanting to develop a new value chain of wastes as inputs; and can supprort technology and processing barriers to maximise potential success (EMF, 2013) To the writers knowledge, NISP has not (yet) helped develop local networks for product cores, the work has been developed more in secondary material markets (see ‘Recycling’ for more details).A) Pre-treatment Waste Sites:
B) Brokers:
C) Dealers: This groups principle purchase and sell waste, however, they do not take physical possession of the waste. This can be a company that is buying and selling, or an internet platform that facilitates the buying and selling by others.
D) Public-Private Regulators: This includes Public or Public-Private groups that manage and regulate the waste management system at a regional, national or transnational level (i.e., Europe for example). These groups, like ‘Eco-systèmes’ in France, can be those who are responsible for the distribution of the ‘Ecotax,’ to those companies working in Revalue activities. Other examples include Zero-Waste, which exists in various forms in many different countries and regions around the world – zero-waste Scotland, is a particularly dynamic example. On the map, this group is also an example of an Action Group, but is also mentioned here, as they are an integrated part of this Waste Management Group, and so, the key link should noted.
This includes the point of collect (which includes a first level of sorting and screening), and the logistics required to move the core/product to be stocked in an independent ‘Inventory’ or the site for ‘Reverse-Engineering.’
For revalue companies to enter into a reverse logistics and collection activity, their has to be an economic incentive for them, and often also for the customer. Prior to giving more insights into the collection process, here are some more details about some of these incentives:
Core Value: What is the value of the Core? In the case of an independent/non-OEM revalue manufacturer, the process can start with the core brokers and the a remanufacturer making a big for a particular part; and supply and demand dictate prices (Schinzing, 2007). As well as market demand, the value is also linked to many factors, such as model, quantity, quality, product-cycle, rate of technological change, and supply and demand for example. See the next section ‘Inventory’ for more information.
Core Acquisition: If the reverse engineering is being made by the OEM or a sub-contractor of the OEM, then they have the possibility to build-in some form of ‘Business Model’ to increase ‘Recapture’ of their own products. To find out more about these types of business models, and their numerous advantages, go to ‘Design for Revalue’ and ‘Business Models.’
There are however, a number of Business Model options for non-OEM revalue companies, when attempting to source cores, and they are described below:
Buy-back: In this case, the remanufacturer simply buys the core. There is no relationship before or after the transaction. (Sundin et al., 2013)
Credit-based: In this case, the customer receives a credit for returning the core. This credit then can go towards a discount when buying a remanufactured product for instance. In this example, the supplier can also be the customer. (see Sundin et al., 2013)
Deposit-based: This is when the customer is obliged to return a similar used product, when they buy a remanufactured product. This is a frequent transaction in the auto-industry, such as brake callipers for instance. (see Sundin et al., 2013)
Direct-order: Here, the customer sends a used core to the remanufacture directly, and the remanufacturer sends a replacement back. The replacement cor can be a like-for-like exchange, whereby a previously remanufactured core is used as the replacement, or the original core is sent back after it has been remanufactured (Sundin et al., 2013).
Reman-contract: This is similar to direct-order (shown above), except here, there is a contract between both parties, determining who the remanufacturer will be, if and when they are required (Sundin et al., 2013).
Collection & Transportation: Here, the product needs to be collected, either directly from the original consumer, or from one of the ‘Core Brokers.’ In all cases, the product has now left its’ working location, and is being transported to one of the ‘Reverse’ Engineering sites and/or ‘Inventory.’
If the core had been through the ‘Design for Recapture’ process during its’ development (see more about strategies for improving Recapture in the sub-group ‘Design for Revalue’), then this stage can be considerably improved.
Firstly, the collection systems need to be user-friendly: they need to focus on the key congeners of the person/company returning a product (i.e., deletion of a user’s phone data for instance); they need to be located close to the customers and the life-extension specialists; and they need to be able to stock and maintain the quality of the goods and materials they have recaptured. (see EMF, 2012)
This part of the revalue process can be critical in the economics of the system, as without cost-efficient, good quality collection and treatment systems (recapture), the economics of the revalue chain is undermined. As EMF (2012) highlights, it is for this reason that the building-up of the infrastructure and its’ capabilities, to close the loops, is critical.
Reducing distances from the collection point and the place of remanufacture, can be fundamental, according to EMF (2012). As they observe that material recycling may be cost effective on a global, or at least, inter-regional scale. However, harvesting of components and other life extension strategies, often only make sense on a local or regional level (as the cost of logistics can be simply too great). USITC (2012) underlines the point for remanufacturing:
“Transportation and labor costs strongly influence where remanufactured goods are produced. Over 36 percent of HDOR equipment remanufacturers and 32 percent of motor vehicle parts remanufacturers cited transportation costs as an “extremely” important competitive factor, reflecting in part the importance of regional production networks and producing closer to the intended market of sale. The costs of shipping inbound cores to be processed and outbound remanufactured goods for distribution contribute to the tendency of remanufacturing to be done close to the U.S. market, favoring Canada and Mexico.”
A typical example of a collection system by an Original Equipment Manufacture is Caterpillar Inc. Caterpillar, which has a global vendor and distribution system, uses it as a collection point and network for most of its cores (engines in particular). And lastly, it should be kept in mind that:
“Apart from the automotive industry, few industries currently achieve a collection rate of 25%. When shifting from linear to circular approaches, the rule of thumb for optimisation is: ‘the tighter the reverse cycle, the less embedded energy and labour are lost and the more material is preserved.” (EMF, 2012)
Pre-Sorting: This is where a simple or more technically refined set of protocols are put in place to judge the quality of the core at the time of collect, and the ‘quality’ during transportation. Sundin (2013) highlights that storage during transportation is also critical to maintain quality.
If the original product had been through the ‘Design for Recapture’ process and the ‘Design for Fault Detection, Inspection & Sorting’ during its’ development, then quality could be better maintained and measured during the collection phase. Highlighted by the EMF (2014):
“Sophisticated reverse network management capabilities are another part of the puzzle, best fuelled by investments in hardware (e.g. sorting and manufacturing capabilities) and software. The latter will need a high level of sophistication, such as materials databases, methods for monitoring the condition of used components, and inventory management tools to store BOM information. Companies working hand-in-hand with governments and industry associations will have the best chance of establishing standards to ensure product quality and supply chain transparency.” (EMF, 2014)
An example of a pre-sorting process of washing machines made by EnvironCom in the UK, according to Tuppen & EMF (2013), is that a when a machine first arrives on the site, it goes through a grading process. Some older products can be rejected (and so sent to recycling) directly. The remaining 35% – 40%, can go through a process of refurbishment. In this process, these products can also be graded into ‘silver’ or ‘gold’ categories, to frame the following level of technical intervention required:
It should be noted that the pre-sorting operation should ideally be made at the customer’s location. It isn’t worthwhile to make the whole recovery process for a product which has no demand. It can reduce costs significantly. Moreover, the reverse logistic and engineering process can be different if the product is broken or if it doesn’t fit anymore the customer needs.
Pre-sorting can be made by the customer, however, Sundin (2013) highlights certain problems, as this can lead to the selection of unwanted or below grade parts.
Pre-Dismantling/Disassembly: In the case of the product arriving at the end-of-cycle, as mentioned in ‘Peddlers’ within the group ‘Core Brokers’, there can often be a pre-dismantling of a product made on site, as the entire product might not be worth transporting to the reverse-engineering site or Inventory. And, in the case of a product which is still in use, the core that is removed, could be a motor, which in the ‘Maintenance’ phase, could then be sent to a revalue company, and replaced by a new one, or a motor that has already passed through the revalue process.
This section enters into more depth in one of the key elements of Revalue, and that is the management of stocks and flows of cores within the system. In this map, the word ‘Inventory’ is used to describe the actual physical place that cores are stored in, to distinguish it from ‘stock’, which can be used to describe the same thing, but also means the amount of cores flowing through the system. Therefore, stock is only used in this map in relation to flow.
Cores can often pass through multiple Inventories, potentially at the various ‘Core-Brokers’, ‘Reverse-Engineering’ companies, and the companies who finally fit the revalue core into a product for example. The Inventory can also be based in a specialised company, dedicated to managing the flow of cores through the system. A specialised Inventory, is also a form of ‘Professional Core Broker,’ like Autoenterprises in the UK, mentioned in the section on ‘Core Brokers.’
‘Cannibalise’ or Cannibalisation, is the process of stocking cores that are evaluated to have an economic value in the future. The cores that can be cannibalised are those that don’t need any reverse engineering work done to them, those that need some form of reverse engineering work done to them in the future, or are those cores that have already passed through the reverse-engineering process.
Cores can be both products (like motor engines), or sub-assemblies, or components (like micro-chips). Sony Europe, with the Playstation, is a classic example of a company that stocked cannibalised cores for a very successful ‘After-Sales-Service’; highlighted here by Parker (2013):
“Over the last 3 years, SCEE has reused over 6.8 million components from both the PlayStation® and the PlayStation2®. These range from screws to expensive processing units and motherboards. These parts would otherwise have been shipped from the Far East, incurring not only purchase cost, but also Shipping and handling costs; instead they were reused predominantly at the centre where they were reclaimed.”
The reverse-engineering company also managing the Inventory, presumed rightly that the sub-assembles, once removed from faulty Playstation units and then stored, would have a value later on, after analysis, to be used again within other Playstation units which had faults corresponding to the same parts. The Playstation example, is an interesting example of an ‘Advanced After-Sales-Service model’, and one in which could be mimicked by many After-Sale-Service departments within OEM manufacturing companies. This particular point will be discussed further, in the main text on this webpage.
These reusable parts, obtained at the various different stages of the reverse engineering process, can represent a large percentage of the components that enter back into the revalue products. This is one of the main economic interests of revalue engineering – as if it were not the case, and a majority of the parts had to be replaced with new ones, then reverse engineering would be completely uneconomical.
Below are a list of four main variables the Inventory has to contend with:
1) Quantity of Cores (and Space): Quantity links directly with point 3 below ‘Controlling Timing of Cores,’ however it is not the same. For example, a company may be able to predictably receive 10,000 cores per year (Quantity), but they may all arrive at different times throughout the year; and so even though the total amount (on paper) seems economically viable, a variability in flow timing can create periods of insufficient labour, or too much for example – making the activity non-economical. Sundin et al., (2013) also highlights that a large number of small batch volumes, increases complexity.
Sometimes Core Brokers may supply a minimum quantity (to make it economic for them), and so a reverse engineering company/department may have to take more cores than it wants, or has clear demand for. On the other hand, the reverse engineering company/department may lack sufficient cores, particularly for fast-moving products.
As with point 3 below, the variables of quantity can be managed to some extent (if possible – but not so easy for non-OEM revalue companies for example) by implementing some of the Business Models based around services (see ‘Business Models’ for more information). And Sundin et al., (2013) also highlights the fact (linked also to quality) that two identical returned products (cores) may require very different revalue processes (depending on their condition), and so inventory plants and cost and purchasing controls can be difficult.
Quantity and Timing also links directly to the space in the stock, and the related costs associated to the loss of space for every core stored, and the cost of maintaining the space. Space management for cores may be more complex than an inventory for new products in a OEM, as cores can vary more in size and shape (cores may come from a range of different manufactures, and include various models), and are invariably not packed, so stacking and optimising storage is not always very easy.
2) Quality of Cores: This depends a lot on the type of product. An example of good control of quality by Sundin et al., (2013):
“[This revalue company] claims to have total control of the quality of the returned brake calipers. There are two reasons for that. Firstly, brake calipers have a simple design and are made out of a robust material but also contain few parts, which make units worn beyond their remanufacturability infrequent. Secondly, because of their design, it is easy to visually determine whether a unit is remanufacturable or not [easy sorting…].”
The continued review of quality and value over time, is also a key activity in the Inventory. It is imperative, due to space and maximisation of the stored assets, that the inventory is constantly monitored (visually, and with a database), and that the parts are used or sent to recycling at the right time. Quality of parts can possibly degrade over time, however, it is more likely that the parts move closer to obsolesce (depending on their ‘rate of technological change’) the longer they are stocked (this may be the opposite in the niche market for collector cars for example, where pieces may actually increase in value over time).
To improve quality “[some revalue companies also use] …check sheets that private customers and car dealerships/repair shops must fill out in advance to avoid receiving non-remanufacturable cores and cores too costly to remanufacture. This improves quality control, but does not eliminate the need for quality inspections at arrival.” (Sundin, 2012)
The handling of cores is also a key concern for maintaining the quality of cores. For example, a core can be in good quality prior to reverse logistics, and then be mis-handled, and damaged (Also see the ‘Reverse Logistics’ section). An example from Sundin (2013):
“Company E estimates that 60% to 70% of the initially sound diesel particulate filters (DPFs) from one of its core suppliers are being damaged when dismounted from the cars or when transported to company E’s remanufacturing facility. The damages are assumed to be caused by the mechanics at the OEM dealers as well as by the companies responsible for the transportation of the cores – they do not recognize the value of the cores and thereby handle them in an incautious way.” (Sundin, 2013)
This can create a huge loss in potential products/cores/components for revalue processes; and instead creating a greater flow to recycling (and potentially landfill).
3) Controlling Timing of Cores: There is often a real lack of control on the timing of returned cores (as mentioned in point 1). Products will of course break or be discarded prior to their end-of-cycle phase, however there is also the ‘product cycle’ to consider. As described by USITC (2012):
“The supply of cores to be remanufactured is limited at the beginning of the life cycle of a new product (such as the introduction of a new model), generally increases as the product type or model matures, and finally declines as the product type or model begins to be withdrawn from the market. During this cycle, warranty coverage tends to increase the flow of cores as buyers opt to replace rather than repair worn-out goods. For instance… when a component of a new engine is first introduced in a motor vehicle, few to no cores are available to remanufacture that part. As the part ages, however, consumers replace the component and return an increasing number of cores, thereby creating a supply of cores viable for remanufacturing. Core availability begins to decline again as the vehicles in which the part is used are removed from service.”
Knowing how high the demand is also, underlined by Schinzing (2007), a critical piece of information. So, a revalue company and/or inventory, should be aware of these supply and demand stages within the product life cycle.
Another way to improve the management of core timing is to create a service (i.e., renting or fee-for-service) style ‘Business Model’ (see the section on ‘Business Models’ for more details). With a fixed term contract, and direct access to the cores, revalue companies can clearly reduce, or even eliminate this variable.
4) Supply and Demand Balance: Here, linked to quantity, quality, and timing, the Inventory has a lack of control of balancing supply; but they also can have difficulties predicting demand. Having a view of demand can depends a lot on who is doing the reverse-engineering work, the type of product, and who the customer is. For example, if it’s a sub-contracted remanufacturer, they may have a 12 month vision of demand, and a clear view of what types of cores and some understanding of the level of work involved. Independent Remanufactures that work directly with factories (i.e., robotic assembly capital goods) or garages (i.e., drive-chains or water pumps) for example, may also have a good view of their customers previous yearly demand – by keeping yearly statistics of demand. Sundin et al., (2012) describes another, more speculative, scenario in which:
“…company F buys certain cores without having a concrete demand but believes it will rise in the future. The motive is twofold; cores that are bought prior to the actual demand are cheap, hence displaying large profit margins if later sold. In addition, buying pre-demand cores prevents or diminishes competition within core acquisition. However, the speculating comes with a price tag. Apart from the obvious cost associated with storing cores until demand arises, there is an imminent risk that the anticipated demand never occurs, thus making the acquired cores less worth.”
Most revalue companies do not focus too heavily on perfecting the balance between supply and demand, simply because it can make inventory management and control functions too complicated. Those revalue companies that do not try and balance at all, have a high scrap rate – sending a a lot of non-economic cores to ‘Recycling.’
This section looks at the systems that can be and are put in place to make many of the product life extension strategies possible: you can not 're' something that you don't have...


The term ‘maintenance,’ is used to describe the work made by industry, at the scales of industry, which includes repairing, (but different to repairing in the market, discussed in the section ‘REMARKET‘), and other work – inspection and servicing. Maintenance can be done by a variety of individuals and companies, during the working-life phase of the product, either at the products location, or in another specialised maintenance facility; or afterwards, at the end of the products’ end-of-cycle phase (post customer waste – waste or used goods). Within ‘maintenance’ the three activities become the different levels of intervention made by a maintenance engineer. For example, a car owner can take their car to a garage for a maintenance check. Here the mechanic will first Inspect the car, to see if there is anything that needs doing, and if so, Service it (replace the oil), and/or Repair a fault if required. A) Inspection: This is usually a process that is made after a defined period of time (i.e., continuous checking by computer systems, or daily or monthly checks by a maintenance engineer for example). Here, the inspection work often involves a procedure (or protocol) to check the performance of the product, to see if all is working correctly, and to see if any further intervention is required. B) Servicing: A simple example of this is an domestic car oil change, made after a defined number of kilometres of use. In a service, nothing is usually broken, it is more the case of refilling liquids (like the car oil example), or changing parts that have a tendency to wear (like car tyres, engine drive belts and so forth). These parts that wear, are known as non-durable goods or consumables (see more about non-durables in the section on ‘Design for Multiple Cycles’). C) Repairing (Specialised and Industrial): The following definitions of Repairing are used in this study: “Repairing is simply the correction of specified faults in a product. When repaired products have warranties, they are less than those of newly manufactured equivalents. Also, the warranty may not cover the whole product but only the component that has been replaced.” (Ijomah et al, 2007) “Repair[ing] makes a broken product operational again. An analysis of the root cause of the problem is generally not performed in the repair process which means the product may not perform like a new product. Typically, a warranty on a repair will only apply to the specific repair and not the whole item.” (Gray et al, 2007) Who does the maintenance work, and where and when it is done, depends on the ‘Business Model’, ‘Re-Marketing’, and ‘Design for Core Collection’ put in place by the Original Equipment Manufacturer or the Original Retailer, or the preference of the Original Customer. To find out more about ‘Repair’ go to ‘After-Sales-Services.’
The following definitions of Refurbishment are used within this map (in some literature, there can be cross-overs with definitions for Renovation or Reconditioning, or this term is not even used):
The, predominately, aesthetic improvement of a product, which can involve making it look like new, with limited, or no improvements to functionality (APSRG, 2014). And another varient on the definition, which involves slightly more focus on reparation work:
“A process of returning a product to good working condition by replacing or repairing major components that are faulty or close to failure, and making ‘cosmetic’ changes to update the appearance of a product, such as cleaning, changing fabric, painting or refinishing. Any subsequent warranty is generally less than issued for a new or a remanufactured product, but the warranty is likely to cover the whole product (unlike repair). Accordingly, the performance may be less than as-new.” (EMF, 2012).
Refurbishment, therefore, invariably involves some form of cleaning, and is often (but not exclusively) done at the products end-of-cycle phase (when it has been disposed of by the original customer). The refurbishment operator, is very often also a re-seller, and so often wants the product to look it’s best, to gain the best possible price with the minimum of technical intervention (labour and tools). Refurbishment, can include cleaning, and no actual in-depth inspection, servicing or repairing, which is what also distinguishes it from ‘Maintenance’ or ‘Recondition’. In some cases, the products are already in very good condition prior to refurbishment:
“[The]…most “used” consumer products are actually goods that have been returned to a retailer because the buyer changed his or her mind, and not because the good in question is at the end of its useful life. As a result, the good may only require a cosmetic refurbishing before it can be resold.” (USITC, 2012)
A significant group of actors that are involved in the refurbishment of end-of-cycle products are Social Organisations, which also often manage their own reverse logistics directly from customers homes, ‘Retailer Sites’ or other ‘Core Broker Sites’ – also see the section on ‘Reverse Logistics’ for more details. Examples include as Envie in France, Emmaüs International), and more traditional organisations like Environcom in the UK.
The definitions of ‘Recondition/Renovation’ used within this map are:
The potential adjustment to components, bringing a product or component back to working order – although, not necessarily to it’s orignal ‘new’ state (APSRG, 2014). And a definition, which includes greater details on the warranty:
“The process of returning a used product to a satisfactory working condition that may be inferior to the original specification. Generally, the resultant product has a warranty that is less than that of a newly manufactured equivalent. The warranty applies to all major wearing parts.” (Ijomah et al, 2007)
Here, is a short overall description by Parker (2007) of the advanced ‘Recondition/Renovation’ model put in place by Sony Europe:
“A full bench test is… carried out on all consoles and any minor repairs are made. Cosmetic parts are cleaned or replaced as necessary. 85% of consoles will leave this process in full working order ready for shipment.” (Parker, 2007) (process form Sony Europe) This is in effect a more ‘advanced after-sale-service’ model.
The different stages within Renovation/Reconditioning can be broken up into five key steps (which can vary in order, depending on the industry/product):
Test: ‘Failure Detection’ is the first test, detailed below; however, there are all types of tests made throughout the process.
If the ‘Design for Fault Detection, Inspection & Sorting’ has been included into the products’ development stages (See the ‘Design for Revalue’ Sub-Group for more information), then the product may have integrated features that make this part more efficient. For example, those who own a relatively modern car, are probably aware of the ‘computer’ that the mechanic attaches to the engine to diagnose faults.
Failure Detection: This can be made onsite, during collection, and/or at the Inventory, and/or at the site where the refurbishment will take place. Again, as highlighted above, features can be integrated into the product so this part can done easier on location, or at a distance via the internet for OEM, Original Retailers, or After-Sales-Services for example.
Specialised equipment and processes are usually used for this process (see ‘Inspect & Sort’ in ‘Remanufacture for more details). This is an example from EnvironCom, a WEEE revalue firm based in UK (taking about fault detection of a domestic washing machine):
“…a full fault diagnosis will… take place. The three most likely causes of faults are the drum bearings, the pump and the circuit board. Problems also arise due to faulty main motor brushes and bearings, wiring, heating elements and leaks. A machine with too many faults will be scrapped.” (Tuppen & EMF, 2013)
Sub-assembly dismantle: This stage includes the disassembly of the sub-assembly that has failed. Also, at this stage, there will be some form of assessment, to plan the actions required to Recondition the core. If this is a sub-contract remanufacturer, then this will also include a phase to generate a ‘Quote’ for the customer.
Repair or Replace Part: This stage includes repairing or replacing the components. Here, the revalue engineer may not have access to the new spare parts (they may be out of issue for example), and so in some cases they may make new parts (which may even be better than the original, so this can be a form of upgrading), or have a stock of ‘cannibalised’ parts in the ‘inventory,’ which they use to replace the failures part.
Sub-assembly Reassemble: This stage is putting the sub-assembly back together again.
Clean: Revalued components are then cleaned prior to being stored (‘Inventory/Cannibalised’ – for later use or sale), or prior to re-integration (reassembly) into a final product. Often, a final test is also made, such as a wet test for washing machines, which includes two separate wash and spin cycles for instance (Tuppen & EMF, 2013).
What happens next depends on which type of company is making the Recondition/Renovation work, and whether the product is a subassembly core (i.e., an engine), or a complete product (i.e., a washing machine).
Remanufacture requires the highest level of skills and industrial infrastructure (which often relates to investment requirements in capital goods, and therefore, often requires large economies of scale), with the highest quality of revalue core or product as the output of the process.
In this map, this definition is used, which was co-developed with remanufacturing.fr (2015):
An industrial process that recaptures the intrinsic value of components (formed/machined materials, energy and knowledge) from end-of-life products; these components are then used to make new products with equal or higher performance, quality and warranty.
Studies of different types of remanufacturing companies have shown that each company organises its’ processes in various ways, as argued by Gallo et al (2012) and Sundin (2004):
“Although the sequence disassembly -restore-assembly appears to be a fixed point, activities such as inspection, cleaning or testing have not an unique position into the process. The sequence, therefore, must be chosen considering the recovery process, the characteristics of the product, and the technology available for treatment.” (Gallo et al, 2012)
“For example, the cores could either be disassembled followed by inspection (e.g. error detection) or the inspection could be the first step, without first being disassembled. In research, the remanufacturing process often is described with the inspection step taking place after the cleaning and disassembling steps. This, however, is not efficient if the product has fatal errors, which make it less meaningful to remanufacture. In addition, the product is easier to inspect when cleaned, and some products might be impossible to inspect if not cleaned.” (Sundin, 2004)
Taking this into consideration, the following list of key stages, can differ:
Test: Different types of testing procedures can be made at each stage of the process. See below in ‘Inspect & Sort.’
Dismantle (Disassemble/Strip): This dismantling of the core, has in many cases followed at least one dismantling phae, if and when the core was removed from a complete product. Dismantling the core down to its key components is one of the unique processes within Remanufacturing, compared to other revalue processes.
And so according to Ijomah (2004) the disassembly of large parts may occur in several stages: first by subassembly and then into smaller components. If the product has not been through the ‘Design for Disassembly & Reassembly’ (see this section for more information) during the design stage, it is likely, as Steinhilper (2000) highlights, that dirt, rust and oil can make this process very time consuming.
However, the main difficulty in disassembly, is that assembly operations, such as joining obtained by pressing, welding, riveting, gluing and forging, have no reverse operation procedure. And so, another task within disassembly is the identification of which elements can not be recovered (such as the riveted sections), and are, therefore, scrapped (Steinhilper, 2000). And so, disassembly can only go to the point where continued disassemble does not irreversibly damage or damage beyond economic sense, the core elements.
Inspect & Sort: If the product has been through the ‘Design for Fault Detection, Inspection & Sorting’ process during its’ development, then this phase can be far more efficient; for example, without any identification information – like codes (RFID, or bar-codes, or numbers for example) it can be difficult or even impossible to identify what the part is, it’s age, material composition, and the OEM; and it is often impossible to gauge the quality of internal components solely through visual information alone, as this is not reliant on the age, make or model.
The inspection and sorting phases are very closely related, as the second activity can be viewed as the completion of the first. And so, the result is the sorting of cores into three subgroup (Gallo et al, 2012):
- Reusable cores, which do not need revision;
- Recoverable cores, which do not need revision;
- Non-recoverable cores.
This part of the process can often require a lot of manual labour, where the worker is sorting between a large numbers of similar, but not identical parts, like screws for example, to classify parts into (at least) these three main categories.
How these these three criteria are defined, is specific to the remanufacture. And the equipment that they use to affordably test these criteria, is also often specific to remanufactures, and is an area of development in itself. Test equipment, is often visual, such as microscopes, high resolution cameras, electronic image processing, and magnifying glasses for example. (Steinhilper, 2000)
Industrial Clean: This particular stage is also unique to remanufacturing, and in nearly all cases, needs specific capital goods (equipment) to complete the process. Many machines have been specifically developed by equipment suppliers, as those used in traditional manufacturing are not appropriate.
The four parameters for the cleaning process, identified by Steinhilper (2000) are:
- Chemical effects (e.g. detergents)
- Temperature influence (e.g. heat)
- Mechanical action (e.g. brushing, and water jet)
- Time (e.g. duration of process).
Cleaning, in remanufacture, is most often used to remove rust, old paint, and grease. The methods used, include those such as: CO2 spray (as a dry ice), and compressed air (Gray et al, 2007), ultrasonic baths, sand blasting, steel brushing, washing in cleaning petrol, baking ovens, steam or hot water jet cleaning, and chemical detergent spraying or purifying baths. Multiple treatments can be applied to the same core, in a sequence or even at the same time.
Rework, Replace, or Upgrade: Now that the parts are ready to be brought back to quality ‘like new.’ This part of the process, usually requires higher skilled labour than those previous. However, most of the time, the work requires standard cutting machinery, such as milling, lathes, drilling, turning and grinding; and can also use non-geometrical processes like hardening, spray painting, powder coating, or galvanising for example. (Steinhilper, 2000)
In some cases, however, some remanufactures have invested in metal spray machines – additive manufacturing, which adds a thin layer of metal to restore worn surfaces, which would otherwise need to be scrapped (Parker, 2007).
Upgrading is not often done, but it can be of economic interest, in particular circumstances. This process can be integrated into, or done after the previous process, where parts (which might still be in good condition) are replaced with parts of superior performance – those closer to the ‘state-of-the-art’ technology. This means that remanufactured parts, cores or products, can be technically as up-to-date as the latest new product coming off the production line.
Levels of automation are nearly always lower (therefore higher amounts of labour), than in OEM plants making original parts, mainly due to the smaller batch sizes in remanufacturing plants, and lower predictability of the work required (therefor increasing bespoke).
Reassemble: This is the process of putting the parts back together again, to reassemble the core or the entire product. This, like the last two processes, needs skilled and trained workers. This is often done, in small batches, on assembly lines, and using the same or similar tools to the assembly equipment used in the assembly of the original product/core. (Steinhilper, 2000)
Final Test: As mentioned in ‘Test’ at the beginning of this subgroup, different types of testing procedures can be made at each stage of the process. However, this is the final test, which can be the same as, or very close to, the tests made on the product during its original manufacture; which usually involved a functional inspection or test run.
A key difference with testing remanufactured parts/cores/products and original parts is highlighted by Ijomah (2004), is that the quality control methods need to be the same or similar to the original manufacture, and that secondly:
“The only difference is that remanufacturing requires 100% inspection because in remanufacture all parts are presumed faulty until proven otherwise.”
This ‘100% inspection’ for each remanufactured part/core/product, is in many cases higher than the inspection rate (which can be random sampling), that is put in place for original manufactured parts. And so, many observers argue that for this reason in particular, remanufactured parts/cores/products can be of a higher reliability than original products.
Reassembled cores may be sold by the remanufacture to the OEM (if they are not the same entity) for its’ integration back into the traditional production assembly line, or put in stock ‘Cannibalisation/Inventory’ – again, maybe their own, or a company specialising in this, or sold either directly to consumers, or to companies who will eventually fit these parts into products already in the market (i.e. a car mechanic, replacing an end-of-cycle motor in a car, with a remanufactured motor).
Maintenance is potentially the smallest loop within reverse engineering, in that it should require the least amount of resources, to extend the life of the product. However, irrelevant of which reverse engineering process is being used, there comes a point that product-life-extension, does not automatically equal reduced resource use over time for the provision of a particular function.
For example, unless some form of upgrade is made, or is possible, then many products are stuck with their ‘designed-in’ levels (Intrinsic Quality & Performance) of required consumable resources (i.e., energy, water, oils and detergents), that are required and used during their working cycles. And so, this resource consumption during use, can both increase over time due to general wear and tear, and increase in comparison to the latest state-of-the-art technologies. And so, when looking at the total resource consumption (LCA):- during manufacture, use, and revalue, there comes a point that it will require less overall resources to provide the function over the long term by replacing the product, than extending its’ life any further. Tuppen synthesises this point:
“Using data from suitable lifecycle assessments (LCAs) it’s possible to calculate the ‘optimum’ obsolescence point. That is the point in time at which it becomes better to replace a machine than maintain it.” (Tuppen & EMF, 2013)
Calculating this point is not easy, but when a product does reach it, then it makes more sense for the obsolete product or core to be dismantled, and after extracting the maximum number of sub-components that can be salvaged (cannibalised) as spare-parts, the rest that is obsolete and un-usable, then passes on to be recycled.
This section looks at the systems and processes the manufacturing industry can put in place to extend the life of products - and recapture value (make a profit), in the process.

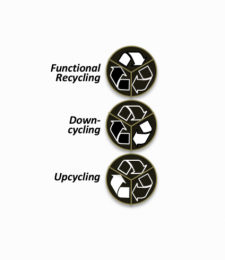
This is, principally, the recovery of used materials, that are then recycled back into the original purpose, or similar purposes, excluding energy recovery (EMF, 2014). A classic example for recycling used materials into the same product, is the collection of used glass bottles, and reusing the glass (after it is processed), and making more glass bottles. This can include, using bottles in their entirety and then cleaning them for reuse, or using the glass and breaking it down, and processing it for reuse as one of the material feedstocks for the manufacture of new glass bottles. However, there are many problems with this form of recycling, as Pauli (2010) highlights: “…nature has some very simple and clear principles and leaves are never recycled into leaves.“ And he continues to explain, that the recycling of glass bottles back into glass bottles is a very complicated task; which includes careful separation of colours (i.e., clear, green, brown), and the polycarbonate plastics found in car front-window-screens (another major source of glass which can be used as a feedstock). See an alternative explained in the next section on ‘Upcycling’. As is discussed in more detail in the section on ‘RE-DESIGN‘ (below) – there are many potential strategies, that can be implemented, for improving the quality of the secondary feedstock prior to reuse. These include, ‘simplifying/purifying’ the materials, and eliminating/managing ‘toxicity’ – during the design stage; designing in efficient processing and separation of ‘technical’ and ‘biological’ materials during used material collection, which includes improving the ability to identify the quality and type of materials more efficiently, for instance. This is, the conversion (or simply crushing) of used materials into new materials of lesser quality and reduced functionality (EMF, 2014). Or, seen from another perspective, it can be a cheaper way to ‘get rid’ of waste than by traditional waste disposal (Pauli, 1998). An example of this, is the use of secondary material feedstock as a cheap ‘filler’ for other products. This is where the actual functional attributes of the material are not used to the full – or at all – and where the lowest price, and how much can be used within the final material without reducing its’ acceptable performance, can be the overriding incentives. An often used example is mixing (cheap) used materials with other aggregates to build road surfaces. Today, downcycling, and functional cycling are probably the most common forms of recycling processes used. This is, the conversion of used materials into new materials of higher quality and increased functionality (EMF, 201) This can, at first seem impossible, or at least, counter intuitive – surely entropy means that materials over time always degrade? However, if one looks at how nature is separating and integrating materials in constant cycles, with no loss of quality, and as these systems have all co-evolved, the previous separating or integrating by another kingdom (or species) is very often critical for another kingdoms existence. Cascading: Here, is a short extract from Pauli (2010), within a description of the concept of the ‘five kingdoms’ (one of the pedagogic taxonomies of life, promoted by some scientists): “Whatever is waste for one species, is a nutrient or energy for another species… belonging to another kingdom.” (Pauli, 2010) There are numerous examples of this theory in practice, thanks in many ways to the work and promotion of Pauli and the ZERI foundation and it’s global network (see the cases described at www.theblueeconomy.org). An example in industry, of upcycling glass bottles (discussed in the ‘functional recycling’ above), is a technique developed back in the 1930s, where glass containers are shredded into dust, and heated up in a oven, which is powered by the combustion of methane gas captured from a local landfill site, and injected with CO2 (which is also captured from the local landfill, as 30% of the gases given off is CO2), that then turns the glass into a foamed glass product (Pauli, 2010). From this example (see Case study 103 from the blue economy), a range of different companies have been created and are developing a portfolio of products, including ‘Earth Stone International,’ a company that currently develops ‘Growstone,’ a growing medium that can be used in hydroponics, and ‘Quicksand’ a sanding block; and ‘Misapor (CH),’ a company producing foamed glass as an insulation for construction, that has both multiple functionalities (i.e., it is also structural, and can be used without an extra aesthetic coating) and also has multiple insulation uses. The Misapor foam glass example, with its’ multiple functionalities underline it as an ‘upcycling’ – increased functionality – process. The example also shows how a mix of different ‘waste’ streams, in this case, CO2 and Methane (two important green house gases), and glass, can be combined to create a high value product. Some of the benefits of cascading can be described through the arbitrage value, which is the potential for the lower marginal costs of reusing cascaded materials than virgin materials (including their embedded costs of labour, machinery, inputs etc); as well as, the externalities against bringing the used material back to original use (if even possible (EMF, 2012). However, cascading is more than an arbitrage opportunity – and luckily so, as this lower cost of used materials is far from a guarantee. Cascading, is also about innovation, and turning an opportunity into a functioning business – and potentially multiple businesses – as cascading can involve a material having extended value within multiple cycles. Cotton clothing (a ‘biological material’) for example, can be reused first, as second-hand-clothing, then as quality decreased beyond functional recycling, the material can be used as a fibre-filler in upholstery in furniture, and after this, as ground up fibre for wall insulation in construction (EMF, 2012). In each of these cases, it is replacing the need to use a virgin material. And as it is a biological material, it can finally go back, via composting, to the soil. At the level of a region, the cascading of secondary materials, may be to some extent, facilitated by intermediates (including some Core Brokers), helping to connect supply and demand. For example in the UK, WRAP (Waste and Resources Action Programme) has played an instrumental role in establishing markets for secondary materials in the UK (EEF, July 2014). Also see more information about NISP (the U.K.’s National Industrial Symbiosis Programme) in the section on ‘Brokerage Facilitators,’ under ‘Core Brokers’ within the sub-group ‘Recapture’, which is an example of a group helping to establishing regional cascading markets. This example has more recently been evolved and developed all around the world, with the association of urban coffee shops (spent coffee grains) and urban mushroom farmers. Once these businesses are both up and running, there will be other secondary outputs, which can then lead to more activities, which can also be connected to other existing local businesses, or created businesses, and so on through a process of autopioeses… This form of cascading, as highlighted by Pauli (1998): “…requires a multidisciplinary approach. It can only succeed with co-operation across business sectors. For example, fibres from the plantation are re-used in the pulp industry: lignin as a binding agent and hemicellulose in the food industry.” This is a system focused on the economies of scope, and so, the symbiotic diversity in the portfolio, can be the resilience of the entire business or group of businesses. Cascading can also help primary producers diversify their portfolio, and also help them move up the value chain towards the final consumer. This can be seen clearer with another example, the coffee plantation: “When coffee is grown, only 0.2 percent of the coffee plant ends up being consumed as an actual coffee; and farmers do not make very much money on that 0.2%. Each cup contains only 3 grams of the biomass that was farmed and is worth less than a tenth of a cent to the farmer. What happens to the rest of that coffee plant? The rest of the coffee plant is unused biomass – actually 99.8 percent of the plant.” (Pauli, 2010) By using the cascading methodology, the coffee farmer can use the waste coffee plant hardwood and/or coffee husks, as a substrate for growing mushrooms, and the remaining spent substrate from the mushrooms can then be used as pig or chicken feed (thanks to the mushrooms, the spent mushroom substrate is now full of amino acids, much lower in caffeine, and the lignin which is difficult to digest has been broken down…). And so, instead of thinking of how the Earth can produce more, we can start to think about how we can start to do more with what the Earth produces. And as Pauli highlights, only 5% of agro-forestry output is effectively put to use, with the rest being wasted (Pauli, 1998); but if 95% of the output can be used, or even 100%, then this is a factor 20 decrease in what the Earth needs to provide. The Biorefinery, has key roots in the field of ‘Green Chemistry’ – to find out more, got to the Royal Society of Chemistry.And so, Upcycling, through this natural systems lens, can best be described through the concept of ‘Cascading’:
Regional Cascading: The concept of dealing with ‘waste’, is now more about identifying the inherent value in secondary materials in a region. In South Korea, for instance, used materials in urban environments, are now referred to as “urban mines,” which have an estimated total value of £29 billion (EEF, July 2014).
Industrial Cascading (Industrial Ecology or Eco-Industrial Park): This is the development of cascading secondary materials at the level of an industrial park. And so, companies with a ‘symbiotic’ supply and demand, position themselves locally together, to take advantage of the reduction in reverse logistic costs (and potentially ‘free’ cost of the input) – which can be a huge hurdle in revalue systems – and more broadly to take advantage of other location benefits and innovations that may arise from being located close together (such as knowledge and trust for example). It can be a risky strategy – after all, this can be a large scale bricks and mortar operation; what if one company up the ‘waste’ chain goes out of business, or changes the output material due to changes in technology? And, having a symbiotic resource chain, does not also mean that the companies are all located in the optimum geographic location for their market. However, the most famous example in the world, the Industrial Ecology site in Denmark ‘Kalunborg Symbiosis,’ which first began over 50 years ago, shows it can be possible, in a particular context, at a large scale.
Portfolio Cascading (Ecological Cluster): This is different from industrial ecology above, as it is typically at a smaller scale, with production close to consumption, initial investments are usually relatively low, and although often involves ecological clusters of more than one business, some ‘portfolio cascading’ can be primarily focused within one business (although always linked to external activities…).
Outputs to Inputs: One example is a brewery and a mushroom farm, whereby, an estimated one ton of mushrooms can be produced on one ton of spent grains (the main waste material from the brewery) (Pauli, 1998).
Biorefinery: To get to a point where all the value is extracted from biological nutrients – the entire chemical breakdown of a material is required – and then the knowledge of how to efficiently/environmentally extract those chemicals, at the right economic quality, and quantity. This type of work is typically made in a ‘Bio-refinery.’ A Bio-refinery can be positioned on the map, within the ‘Transformation Industry’, and as a ‘Recycling’ company. See the Processum Biorefinery in Sweden as an example bio-refinery. Like Industrial Ecology companies, a biorefinery can take secondary material from the ‘Post Consumer Waste’ stream (the brown arrow coming down from the top on the map), or from the ‘Pre- Consumer Waste’ stream (shown as the orange arrow on the map, coming in from the left).
This section looks at the systems and processes available to extend the life - and this case the value that can recaptured from waste or secondary materials.

This strategy has strong links with the ‘Business Model,’ and ‘Design for Fault Detection, Inspection & Sorting.’ The collection phase has many uncertainties for the company recapturing used or waste goods. Companies need to manage the flows of the goods, such as where are they, who has them, when will they arrive, at what price, in what quantity, and at what quality for example… These are all examples of the variables companies have to manage with, when trying to economically recapture these goods. Gray et al., (2027) underlines the fact that revalue cannot occur without the waste/used goods/components/materials to revalue! And so this makes this part of the process critical for the following steps and a successful revalue system. Information can be marked (printed or engraved/embossed for example), on the outside or inside of the product or packaging, with the use of symbols (standard codes, Braille, infographics…), graphic representations of data (barcodes, QR codes…), or RFID tags for example. The style, type, and positioning of the information, will depend on the intended viewer. The collection method can also be communicated in some way, by the actual form and weight of the product itself. For example, perkins engines have code marked on their engines, which show the place and year of manufacture, and the engine type (Gray et al, 2007). Panasonic for example, has developed with RTL (Round Trip Logistics), an online database that can be used by some of the different actors involved in the ‘Recapture’ process, particularly retailers and after-sales-services. In the case of a retailer (who often has to manage a vast range of different brands), when a customer brings a Panasonic product back for refund, or repair, with a particular warranty, the online database provides the retailer with Panasonic’s policies and rules, and the instructions for the following return procedure(s). As highlighted by Gray et al., (2007) collection strategies can also be integrated with the ‘Business Model’ already in place, which includes incentives and communications that ensure that collections are carried out effectively. By using integrated sensors (see ‘Fault Detection, Inspection & Sorting’ for more details), which can be combined with wireless or ethernet cable connections between the product in use and the OEM (or service sub-contractor), the collection of the product can be directly linked to the monitoring of the products/components performance. For example, Rolls Royce (‘Power-by-the-hour’ service) embeds sensors into its’ products, which can anticipate the maintenance of aircraft engines and reduce dramatically on the ground repairing processing time; by knowing when and where to collect the product, and if there is a fault which type, or if the product/component has reached a specific number of cycles, whether it needs to be replaced or reverse-engineered for example. ‘Recapture’ data, can be treated with the rest of the resource data, which may be already being collected, stored, managed, and interpreted by the OEM, sub-contractor, or Independent reverse-engineering company, through the company’s ERP (Enterprise Resource Planning) system. ERP systems, can show in real-time the main business activities, including product planning, manufacturing or service delivery, marketing and sales, inventory management (which is a key element of recapture), and shipping and payments for example. EMF (2013) suggests some other strategies for designing packaging for reuse, such as increasing durability, such as multiple use design (i.e., thicker walls, anti-scratch). And: “Updating current production, transport and retail infrastructure to process reusable containers at scale (e.g., back-haul collection of used containers, washing, refilling).” The packaging, in some cases, maybe stored by the user. In this case, the package is usually specifically designed for the product, so it can be optimum for protecting the product, but it will take up storage space. Or the packaging maybe sent/taken to the user when the product is being recaptured. In this second scenario, the company managing the recapture also has the issue with space, and so perhaps more likely is the use of modular, or adaptable packaging, which may compromise protection of the product.Information: A company can improve ‘Recapture’ of it’s products and components at the end-of-cycle/use phase, by sharing certain information to different actors involved in the ‘Recapture’ process. Some examples:
Systems Techniques: This information, can also be stored within a digital database (similar to the ‘Material Passport’ – See the sub-group ‘Recycling’ for more information), which can also be accessed via barcodes, QR codes, or RFID tags for example, on the product.
Packaging: The product packaging can be designed to guarantee the best conditions to quickly collect and select products, while preserving its’ technical and aesthetic properties, and as mention above, packaging can also be a way to transmit recapture information. For example, not using black coloured materials, as they cannot be detected by near-infrared equipment, or avoiding large labels as they can be mis-detected as actual packaging materials (EMF, 2013).
This is ultimately about designing products/components that can be efficiently disassembled at low costs, whilst preserving their initial properties (high quality) – prior to relevant reverse engineering process(es); and designing products/components that can be efficiently put back together again at low costs, whilst preserving their initial properties (high quality),after the reverse engineering process(es).
Disassembly
According to Gray et al., (2007) there is a long list of reasons why disassembly can be difficult: including, corrosion and rust, dirt, oil, debris, fixed or worn fastenings, complexity, damage (i.e., that reduces access), size (dimensions and weight), tight tolerances, and the requirement for special tools, for instance.
Materials: Selection of materials can help disassembly. For instance, Chiodo (2005) proposes that dismantling times can be reduced (or even eliminated) by making the product or sub-components of the same or similar materials, and with compatible fixings or attachments. This is particularly relevant if there are non-durable parts that need to be removed, which then can be quickly sorted, and efficiently recycled.
Simplification: Standardisation of mountings, reducing the number of components, easy to split materials, uses of common tools… are amongst many other design strategies. Also according to EMF (2012), increased modularity, reduced use of adhesives and increased quality of parts that need to resist disassembly, can also improve disassembly performance.
Active Disassembly: Chiodo (2005) explains that this can be through the use of, so-called, ‘smart materials’ that undergo deformation under specific temperatures; therefore assemblies can actively disassemble without the need of tools or machines to undo mechanical fastners.
For example, materials used for ‘active disassembly’ include Shape Memory Polymers (SMPs) or Shape Memory Alloys (SMAs); and are often used as rivets, bolts or screws, which change shape at specific temperatures (approximately between 65ºC and 120ºC), releasing two or more parts from the assembly.
Fasteners: Active Disassembly, already highlights how good fastener design can improve disassembly, however they should be minimised in number and variety within an assembly (without compromising the structural properties). There should also be a preference of snap-fits over fastners, taking into consideration the general wear (work-hardening, fracture…). If metallic fasteners are used, ferrous types can help magnetic separation; and finally, the access to the fastener is also important.
Also, the minimal use of riveting, as although rivets can be far easier to disassemble than welds, they can still prove very time consuming (Ijomah et al, 2007).
General Principles: This is a list of further general principles developed by Chiodo (2005):
- “Minimise the number of components used in an assembly, either by integrating parts or through system re-design.
- Separate working components into modular sub-assemblies.
- Construct sub-assemblies in planes which do not affect the function of the components.
- Avoid using laminates which require separation prior to re-use.
- Avoid painting parts as only a small percentage of paint can contaminate and prevent an entire batch of plastic from being recycled.”
Reassembly
Designing for disassembly and reassembly is not the same thing. We all know how easy it can be to dismantling things… but the skills required to put things back together again, like in the assembly of a new product, needs to be much higher, and workers also need to have some minimum knowledge of the product(s) and components, and how they work.
According to Gray et al., (2007), it is actually the lack of skills required for reassembly, that is the greatest constraint in the electrical rebuilders section of the automotive industry for instance. Therefore, this suggests the needs both for greater training and simpler, and more intuitive, products to reassemble.
Beyond the skill of the workers, the ‘fastners’ technology – screws and threaded holes for example, need to be robust enough for re-assembly: often plastic screw holes, with metal screws can be defective after they have been dis-assembled only once, whereby the metal screw has destroyed the threaded hole, for example. Also, special tooling maybe required to assemble certain components (which may not be available), and some components may need to be tightened to specific tolerances (which may not be indicated). Finally, the product diversity and complexity, and too light duty materials, can also make reassembly difficult.
Design for Inspection, Fault Detection and Sorting Products/Cores/Materials, are all key strategies in improving the speed of analysis, and the quality of intervention option selected (including the option for no-intervention, or one of the reverse engineering processes) by the potentially numerous actors along the Recapture and Reverse Engineering Process.
In other words, the inspection process, is used to establish the current status and the history of the products/components, to be able to apply the appropriate methods for remediation (Gray et al, 2007).
These three different actions, have been grouped together, as they are very much inter-related: Sorting, needs some information, which can come from some form of Inspection or Fault Detection for example.
There are potentially many Inspection, Fault Detection and Sorting actions made along the Recapture and Reverse Engineering process. These actions can include the analysis and selection of particular end-of-cycle products, during the ‘Reverse Logistics’ phase, which can often be a relatively quick selection of those products that are seen as economically interesting; this ‘pre-sorting’ then leads to progressive analysis and selections, during the products potential disassembly of its’ sub-assemblies and its’ sub-components.
Below, are some of the Design strategies that can be implemented for this part of Design for Revalue:
Materials: Make it easier to identify what the product is made of, and the grade and structure of the material, as this also changes how it can be machined or recycled for example. Ijomah (2009) suggests to reduce the number of different materials used for parts, which can ease sorting; and can also potentially reduce the number of cleaning procedures (the same cleaning technology can often be used for the same materials); and to select materials that can survive the inspection process.
And EMF (2012) underlines:
“Understanding all the materials and components with every product and better labelling of these will be will be crucial for our success in the Circular Economy game’. This information needs to feed into well-developed company-internal databases and tracking systems, so that one can easily look up the origin, age, and range of potential applications…” (EMF, 2012)
A Product Passport or Environmental Product Declarations (EPDs), can also be made available for the Recapture and Reverse Engineering actors, which is a document that describes the materials used inside the product, and can also provide an understanding of the economic value of the different materials, and potential ways to reuse them and manage them. The Product Passport can be made available as an online platform, with varying levels of openness.
APSRG (Dec 2014) highlights how this kind of information can help the actors involved in Recapture and Reverse Engineering (although they focus on the non-OEM remanufacturers, it can still be relevant for all the processes and actors); as this information can help companies know the quality before they collect and buy the object(s).
Product Structure: The physical structure of the product and its’ components can also be designed in a way, that makes Inspect, Fault Detect and Sort more efficient and effective. Ijomah (2009) suggests to arrange the components, so that separation joints are identifiable and easy to access; and to increase the standardisation of components fits, to reduce the number of joints, and the number of parts and part weight. Ijomal (2009) also advises the use of built in handles for bulky or heavy goods.
Ease of access to both the information and the components (which is linked to ‘Design for Disassembly’) is also an important design criteria, in part noted by Tuppen & EMF (2013) that washing machines would be designed, firstly with wash cycle counters (to help in Inspection, Fault Detection and Sorting), and secondly with easy access to the cycle counters. Ijomah (2009) recommendations go further, in that the level of disassembly required to effectively test components should be minimised; and required testing complexity should be reduced and standardised. Goods should also clearly identify their optimum tolerances, and load limits for example.
Linked to access, is the clear marking of where inspection points are, and it is also useful to mark clearly on the product (and/or within the Product Passport mentioned above) the make, model, and year of manufacture. (Ijomah, 2009)
Technology: Different technologies can also be embedded in the product, to facilitate Inspect, Fault Detection, and Sorting; which can be linked to the Product Passport database mentioned above. Such as those outlined by Gray et al., (2007):
- “Embedded data recording, or RFID which may be updated during product servicing etc.
- Sacrificial parts. The application of sacrificial aspects to a component, which would wear during use and thereby give an indication of the components’ treatment over time.
- Traditional data recording of the products’ multiple life history. Xerox for example keep accessible data of all their products previous lives.”
EMF (2012) also discusses how technology can be used to facilitate tracking of products and materials through the value chain, and this may increase with IoT (internet of things) technologies:
“The ‘Internet of everything’. …This interconnectedness will enable tracking efficiency that was previously inconceivable.” (EMF, 2014)
Technology, can also be used to help identify the condition and a fault, with the inclusion of fault-tracking software. The software can identify which part of the good is broken, which highly facilitates the repairing and sorting processes. RFID (Radio-frequency identification (RFID), can also be used to improve sorting, as it is able to contain a relatively large amount of information in a small foot-print, at low cost, and can be read (digitally) at distance and at high speed.
Designing the product and components to be easily cleaned is also one of the key design criteria that can be worked on within ‘Design for Revalue.’ Products and parts are often cleaned for aesthetic (i.e., ready for re-use, or re-sell) or functional reasons (i.e., a buildup of fine particles, in an area that can cause or increase wearing or create a malfunction), throughout the different Reverse Engineering processes; and industrial cleaning is used in Remanufacturing to remove paints, rust and other elements so that other stages of the process are made possible – such as Dismantling, Inspect & Sort and Reassembly for example.
According to Sundin in his study (2007) at Electrolux Motala plant, the step that needs most improvement is cleaning:
“Cleaning is the remanufacturing step that needs to be improved the most according to the data collected. To increase efficiency in this step, the following actions can be taken:
- Install steam cleaning.
- Train personnel so that they become more task-flexible, i.e. personnel from other work areas can ease the cleaning step by doing some kind of pre-wash when needed.
- Design products that do not collect dirt in the first place.” (Sundin, 2007)
Below are some of the different ways in which the product can be designed for more efficient and effective cleaning and industrial cleaning.
Materials: Ijomah et al, 2007, highlight some strategies for the design of the material surface, and some material selection criteria:
- “Corrosion resistance. However, this will depend on the materials as some coating materials may peel leaving debris that may damage components.
- Non-adhesive surfaces. However, it may be difficult to maintain the integrity of such surfaces.” (Ijomah et al, 2007)
Ijomah (2009) adds that the use of stickers should be avoided (as they can detach during cleaning), and avoiding the use of materials that are difficult to clean (i.e., pitted surfaces); and that reducing the different number of materials can also reduce the number of different cleaning agents – or by making the different materials easy to sort (with good ID) can make different cleaning procedures more efficient.
Product Structures: Here are some design strategies for designing the product structure from Ijomah (2009):
- Easy access to all areas that need to be cleaned;
- Reduce the ability for dirt to accumulate (such as grooves, or tight corners);
- Reduce part weight – and therefore increase ease of handling for cleaning;
- Handling can also be improved with the integration of handles;
- Ensure markings on products can withstand cleaning.
Disassembly: Ijomah (2009) also identifies that the the product should be designed so that the minimum level of disassembly is required to clean all the components.
This is all about designing for multi-loops. This asks the design engineer, to design for more than one life cycle, instead of just one. This is perhaps one of the most important and probably the most complex strategy within the products ‘Design for Revalue’; though it is also probably the area that can create the greatest rates of revalue at the end-of-cycle.
As Sundin (2004) mentions when discussing Remanufacture, that is the main goal of designing for remanufacture is designing for part reuse. If the part can not be reused, then cleaning or testing is irrelevant.
Products are very often made up of very different types of components, with potentially different life-cycles, and where some parts can be highly worn and other parts can be virtually unused. Understanding, during the design phase, which parts, at the 2nd or 9th cycle (for example), will likely need replacing (and therefore recycling), can be reverse-engineered (and to what level), and or can be upgraded; and then designing the product and its components with this mind, is the main goal of multi-cycle design. As Gray et al 2007, reiterates, within the same product, different parts need to be designed for one use, or three cycles, recycling, disposal, or nth uses, for instance.
“Less product complexity and more manageable life cycles. Providing stable, sometimes reusable product kernels or skeletons, and treating other parts of the product as add-ons (such as software, casings, or extension devices), enables companies to master the challenge of ever-shorter product life cycles and to provide highly customised solutions whilst keeping product portfolio complexity low.” (EMF, 2012)
Design for Recycling
Inevitably, not all the products, components, and sub-components will be able to cycle through the complete reverse-engineering process, and be integrated back into working products (especially not indefinitely). Some parts will be either too damaged (particularly non-durable goods), or technically obsolete for example, and so these elements will have to be prepared for ‘Recycling’.
Braungart, EMF et al. (2013) highlight that it is important to distinguish between ‘renewable’ and ‘recoverable’ when talking about recycling. And so, when evaluating if a material is ‘renewable’, the level of ‘recoverability’ of that material at the end-of-cycle, should also be evaluated. This ‘recoverability’, and therefore its recyclability can be improved through some of the following strategies:
Simplify (Standardised Pure Cycles): As explained by EMF (2014), the simplification of the number and type of polymers/monomers used (across industries) can increase the economics of recycling.
However, during the last decade, the tendency has been in the other direction. And so at the end-of-cycle phase, there maybe an incredible mix of different materials within one product or multiple products, that are difficult to separate. Chiodo (2005) suggests that this wide variation and mixing of materials (composites) is one of the major barriers in economic recycling (extracting good quality, and pure material streams, at a good price) of vehicle parts (i.e., instrument panels, headlight clusters).
Additives are also mixed into polymers to improve the functionality, and/or to reduce the price. There are potentially thousands of different additives in use, and the problem comes when they render plastics technically and/or economically unviable for recovery. And it not just the issue of what type of additive is in the polymer, it is also how that type of additive has been mixed into the polymer in the first place. EMF (2014) highlights that mechanically mixed polymers are easier to separate than chemically bonded polymers; and that economies-of-scale solution, may help, what is arguably a variety of economies-of-scale problems.
EMF (2014), also goes onto suggests a number of industrial sectors that could be improved in this area, including the paper and card board and polypropylene industries.
Chiodo (2005) outlines some pragmatic ‘simplifying’ guidelines when designing for recycling with metals:
“Unplated metals are more recyclable than plated ones.
- Low alloy metals are more recyclable than high alloy ones.
- Most cast irons are easily recycled.
- Aluminium alloys, steel, and magnesium alloys are readily separated and recycled from automotive shredder output.
- Contamination of iron or steel with copper, tin, zinc, lead, or aluminium reduces recyclability.
- Contamination of aluminium with iron, steel, chromium, zinc, lead, copper or magnesium reduces recyclability.
- Contamination of zinc with iron, steel, lead, tin, or cadmium reduces recyclability.”
When looking at the material, from a complete product point of view, other strategies can be used. For example, using plastics with enough difference in their specific gravity (i.e., greater than 0.03) can help during the floatation separation process of different components; or using magnetic with non-magnetic materials, so magnetics can help separate quickly one element or material from another.
In summary, the benefits of simplifying/purifying the material used in products are that the collection and redistribution efficiency is increased, whilst maintaining quality, which can, in turn, extend material longevity (EMF 2012).
And to lead to the next point, ‘Pure’ does not necessarily mean ‘Non Toxic.’
No Toxic Materials: At the level of the product manufacturer, this is often about companies developing their own ‘black list’ – a list of restricted materials not to be used in their products (EMF, 2014). At the level of ‘Heavy & Light Transformation Industries,’ who create the materials for industry, this can be more focused on designing out contaminants (e.g., colourants, plasticisers, and stabilisers) (EMF, 2013) …and other types of additives (go to ‘Purify’ above for more details).
Technical & Biological: It is important to understand the differences between ‘consumable’ and ‘durable’ products and components. Consumables can be water, oils, chemicals, textiles and biomass for example; and Durables can be computers, engines, metals and most plastics for example. Illustrated by the Cradle-to-Cradle team, they suggest that the majority of consumables should be made of ‘biological’ materials (which is not the case today); and the majority of durables should be made of ‘technical’ materials (which is mostly the case today).
The goal then, is for the biological materials (also called ‘biological nutrients’) to be designed to re-enter the Earth safely, whilst technical material (‘technical nutrients’) to be designed to circulate at high quality without re-entering the Earth’s natural cycles (EMF, 2012).
The biological nutrients can also be designed to ‘cascade’ through a variety of different applications, to extend and diversify value potential, prior to re-introducing it back into the biosphere. This biological cascading concept has its routes in the Blue Economy. Cascading of some of the technical materials is also possible, which is strongly linked to Industrial Ecology. These types of cascading strategies can actually be designed into the product during its’ initial development. See the sub-group ‘Recycling,’ Upcycling’ for more information.
The majority of the technical nutrients, beyond cascading and recycling, are cycling through the reverse engineering cycles; reducing the flow of materials to Energy Recovery or Landfill.
Material ID: This is a strategy to improve the identification of the material(s) at the end-of-cycle phase.
An identification showing what the material is in general, should be marked with the appropriate standard (i.e, ISO 1043), to make identification simpler. However, particularly with metals, the grade of the material should also be shown (low grade or high grade steel for example). If the grade is not shown, then high grades can be mixed in with lower grades, reducing he overall quality potential of the material that can be recovered, and therefore its economic value.
Product Passport: See the section on ‘Design for Disassembly & Reassembly’
Leakages: This is focusing on strategies to reduce (or stop) ‘leakages’ of biological and technical materials. Bio-material leakages for instance, is when a ‘cascade‘ opportunity has not been put in place, or contaminates prevent the opportunity. Technical nutrient leakages for instance, refers to the missed implementation opportunity of a ‘closing loops’ – such as – life-extension or recycling strategy (refurbish, renovation, remanufacture, recycle…) of energy, materials, and components/products (EMF, 2014).
Design for Upgrade
Design for Upgrade means designing in the possibility to upgrade parts and/or software or a end-of-cycle product during the reverse engineering process. Upgrade can mean replacing a part and/or software with more efficient and/or have more modern aesthetics than the original design for example, or in the best case replacing old parts with the state-of-the-art technology of the latest comparable products on the market.
“The increasing rates of technological/aesthetic change in the consumer markets, make design for upgrade particularly relevant to remanufacturing in this sector. Upgrading product function to meet customer requirements can prolong the functional life of products (Sundin, 2004).” (Gray et al, 2007)
This is particularly difficult for independent/non-OEM reverse-engineering companies, that may not have access to the required engineering data, and the latest technology to make an upgrade, although some innovative companies do make their own upgrades and even sell them to the OEM!
Revalue Production: This is the design of the physical industrial space, where the Reverse Engineering actually takes place.
The work space for Reverse Production can be designed and continuously improved, using many of the different process management methods already developed for the linear production system. Some of the more well known, such as Lean Manufacturing (particularly useful for relatively new production setups), and Six Sigma (perhaps more effective when applied to more mature production systems), are already used in Reverse Production design and management.
Lean Manufacturing: is a systematic method for the elimination of waste (“Muda”), within a manufacturing system (wikipedia). In essence, Lean Manufacturing focuses on what adds value, and reducing everything else that doesn’t. Waste in the case of “Muda”, is more than waste of Raw Materials; it also focuses on wasted time of labour (a worker waiting for example), or overproduction (ahead of demand), defects, and wasted transportation for example. In Lean it is deemed a waste, if an activity doesn’t add a tangible economic value to the final product.
Six Sigma: is a set of techniques and tools used to improve processes within the entire organisation. It focuses more on the quality of the output, by reducing defects and minimising variations in the production and business processes (wikipedia). The areas where six sigma are used, can include After-Sales-Service (both customer relations and the re-engineering area for example), and looking at pollution reduction, and increasing profits.
4R (Remove, Reduce, Reuse, Recycle – including Biodegrading): In this map, 4R is primarily focused on the production value chain, and revalue chain, and applying 4R in the use of all the different ‘Raw Materials’ inputs (Abiotic, Biotic, Water, Energy & Land Area), which are imbedded in the infrastructure used for production (i.e., capital goods), imbedded in the products themselves, and imbedded in the ‘waste’. 4R can be a constant, iterative process, much like, and linked to, Lean and Six Sigma for example (discussed above), which ‘reduces’ the inputs required, through increases in efficiency, reusing ‘waste’ raw materials in the production process directly (maybe not for the same function), which again reduces the need for external inputs, and finally by managing those ‘wastes’ that can not be used on site, to be ‘recycled’ onsite or by an external specialist.
4R is an important part of the sustainable use of natural resources, but it is not the complete solution. As highlighted by EMF (2012) through a distinction between efficiency and effectiveness:
“Efficiency vs. effectiveness – a key distinction. Eco-efficiency begins with the assumption of a one-way, linear flow of materials through industrial systems: raw materials are extracted from the environment, transformed into products, and eventually disposed of. In this system, eco-efficient techniques seek only to minimise the volume, velocity, and toxicity of the material flow system, but are incapable of altering its linear progression.”
This first part focuses on the strategies which can be taken by a revalue OEM.
Some companies foresee, or may even experience some market competition between their own revalue products and their own new products (market cannibalisation). In most cases, this can be managed by Grading the revalue products, in terms of price and performance, and selling them cheaper (i.e., -30 to 40%, or -10 to -15% depending on the type of product) than the new product equivalent. Even with this reduction in sale price, it is estimated that due to the potential savings on material goods inputs and services, companies can make an average of 30% EBITDA (Earnings before interests, tax, depreciation & amortisation), compared to the 16% EBITDA average margin earned when selling new products (according to Lavery et al., 2013).
Lower prices can extend the market: Selling a revalue product at a lower price, can attract new customers to a product and/or brand, that normally can not afford it. Here, existing customers may also purchase the revalue product, or they may stay with the perceived level of quality that they are used to (the new product). In this last case, the market has expanded (product/brand exposure is increased to more customers), and cannibalisation of new product sales is reduced or even non-existent.
There are further strategies that an OEM can take to bolster ‘Grading’:
Market Cascading: One way to clearly differentiate between new and revalue products is not to sell them in the same market (Ijomah et al, 2007).
The basic principal is that the lower cost revalue products are sold to developing countries, where it is suggested that the higher performance (and therefore higher cost) is not justified: i.e., a revalued high-speed train or carriage, may not have the infrastructure to be able to run at high-speed in a developing country, so the performance requirements are lower. In the case of ‘Remanufacturing,’ the revalue product may be equal or perhaps better in performance than a new product, so the issue of reduced performance may not always exist. However, this strategy can run the risk of continuing a cycle typical in the second hand car industry, where products cascade down through poorer and poorer countries, potentially competing with local manufacturing, and increasing the density of high consumption and high emission products (old cars with old technologies) in one place (increasing energy demand and pollution for example).
Sub-Brands: Grading can be done through price, and with the use of branding and communication. For example: Siemens has a sub-brand called ‘ecoline’ for revalue high-end medical scanning equipment (i.e., MR Magnetic Resonance machines); and Ricoh has a sub-brand called ‘Green-Line’ for its’ revalue photocopiers for example. The fact that the product has been reverse engineered by the OEM (or by its’ sub-contractor), gives a ‘stamp’ of quality transmitted to the consumer.
These last two strategies can be used by a OEM, and a non-OEM revalue company.
Quality Label: Some companies also use quality labels that are communicated to the customer (beyond that of the brand) as a form of ‘seal of trust.’ Siemens has ‘Proven Excellence’, GE has the ‘GoldSeal: Grow with quality,’ and Philips has ‘Philips Diamond Select: Refurbished medical imaging equipment.’ To date, there are no international, national, or even industry level quality labels for revalue products.
Warranty: One of the differentiations of quality, particularly used between refurbished and remanufactured products is the warranty, as Parker (2007) argues that the warranty can persuade customers that a revalued product is as good as a new one:
“The ‘as new’ warranty reassures customers that they will be buying a quality product with excellent after sales support should they need it. Strict quality procedures ensure that the customers experience is trouble free, building trust and confidence in remanufactured products.”
A warranty can be a strong signal to the customer (primarily as it has economic consequences for the company) that a company has confidence in the quality of their own product, and the reverse-engineering work that has been made on it. The level of the warranty depends on part on the company, and on part on the type of reverse-engineering process made; for example a warranty can be given for the entire product if it has been ‘Remanufactured,’ and for a subassembly that has been fixed/replaced in the case of ‘Reconditioned’ product for example. A warranty is less typical with ‘Refurbished’ goods, usually as the intervention work made is often minimal, and so are the resale margins.
Return-to-market Speed: As mentioned briefly before, different products can have different margins, which can depend on the obsolesce level of product. For example, a laptop has a relatively short life in the market (i.e., 5yrs), compared to a washing machine (i.e., 10yrs) or car (i.e., 13yrs) for example. And so, the potential resale market price falls far quicker over the same period of time for a computer than for the other products mentioned above. It is very important, therefore, if the revalue process is to be economically viable, to make the entire ‘Recapture’, ‘Reverse-Engineering,’ and ‘Remarketing and Re-selling’ processes as quickly, and efficiently as possible – in particular for those products, like the computer (or mobile phone). In the case of the computer the resale price is consequently around 10% and 15% cheaper than the equivalent new product on the market (who’s sale price is falling); whereas, a washing machine may resale between 30% and 40% cheaper than the new equivalent product (who’s sale price is more stable). (based on figures from Lavery et al., 2013)
OEM and non-OEM Competition: When revalue products are entering into the market from a non-OEM revalue company, market cannibalisation can be more difficult to manage for the OEM. In this case, the OEM doesn’t control the amount of revalue products on the market, the quality or the price. And so, potentially they can be competing with their ‘own’ products on the market, which can be potentially below the standard that they would like – which can damage their brand. This is mainly why, non-OEM revalue companies, which are an important part of the revalue ecosystem, and OEMs are not always ‘in phase’ with legislation and industrial policy for example. If these two groups are facilitated to make closer partnerships, then perhaps win-win strategies can be found, and profits, and the real and perceived quality of the product/brand can be maintained or even enhanced. However, according to USITCS (2012), some independent printer cartridge remanufacturers, for instance, claim that OEMs engage in various tactics, such as discrediting the quality of third-part revalue products, or making parts more complex, or promoting direct sales and exchange with customers (effectively blocking third-parties out of the market).
Other strategies to increase selling price, and/or reduce costs of revalue processes are discussed further in the next section.
“One of the key challenges currently experienced with successfully moving to a circular economy revolves around business models. The majority of organisations and companies currently still operate on linear business structures, which transfer producer responsibility for products to retailers or consumers upon their sale. Developing business models in which manufacturers acknowledge their producer responsibility is vital for moving to a more circular economy.” (APSGR, Dec 2007)
Below are a list of some of the business models already used around the world, to improve the efficiency and economics of revalue activities, and to help ‘close the loop.’ They can be used with B-to-C (Business to Customer) markets, and from B-to-B (Business to Business) markets – like the ‘Capital Goods Industry’ for example. To understand more of the possibilities than those listed below, look at Product-Service-Systems and the Performance Economy.
Renting not Selling: Stahel & EMF (2013)
“Selling performance, results, utilisation, services instead of goods means that economic actors:
- retain the ownership of goods and embodied resources; and,
- internalise the cost of risk and of waste.
By comparison, the industrial economy maximises its profits by externalising the cost of risk and of waste. After the point of sale, it offers a warranty for a limited period of time and limited to manufacturing defects.”
This is a strategy that can benefit both the OEM/Original Retailer (and even some non-OEM revalue companies) and the consumer. If the OEM/Original Retailer rents a product to a consumer, then the ownership and therefore the responsibility and risk of disfunction stays with the OEM/Original Retailer. The benefits to compensate for taking on this increased responsibility and risk, is that the manufacturer/seller can better manage a closed loop between themselves and the customer, improving efficiency, costs, and reducing leakages of their products beyond their revalue chain (increasing ‘recapture’ rates). The extended responsibility by the OEM in particular, motivates the manufacturer to start thinking more in loops – and potentially improving the quality of their products and how they measure performance and use – therefore, integrating some of the ‘Design for Revalue’ design solutions. The OEM/Original Retailer now also has a longer service relationship with the customer, which they can be used to build a better relationship and understanding of their needs – as highlighted further by EMF (2012):
“Improved customer interaction and loyalty. Getting products returned to the manufacturer at the end of the usage cycle requires a new customer relationship: ‘consumers’ become ‘users’. With leasing or ‘performance’ contracts in place, more customers insights are generated for improved personalisation, customisation, and retention.” (EMF, 2012)
One of the barriers to this business model is that the OEM/Original Retailer has to cover the upfront cost of the product, and has to wait for the full price to be paid over an extended period, therefore they face a cash flow mismatch. EMF (2012) suggest that this gap could be filled by a financial intermediary.
The advantage for the customer is that they have access to product at a far lower initial cost, and as it is a service contract then the choice and convenience can be tailored over the duration of the relationship, and customers are able to stay up-to-date with the latest technology (including energy consumption, emissions, and safety).
Pay per use/ Fee-for-Service:
This is also a form of service contract, however it goes beyond renting a particular product, at a particular price, per period of time; instead here the OEM/Original Retailer charges for a defined level of performance – and so they are motivated to provide the level of performance, using the most efficient technologies (within the economic limits of the contract), which can also evolve over time, as it is them that is financially responsible for the use cost (i.e., the consumption of consumables, and the wear of durables):
“Rather than simply making a stand-alone sale, the ‘servitised’ OEM provides the customer with certain performance outputs. This model guarantees a result instead of a product: hours of flight instead of a jet engine, full print services instead of a printer, or whole flooring services instead of carpet tiles. It is similar to leasing a product, but goes a big step further as the client doesn’t simply pay for the use of an asset, but for the desired output.” (APSRG, Dec 2014)
The service which is actually provided to the customer depends on the product(s) and the company; however, it would generally include an installation, maintenance throughout the contract, and at the end-of-cycle, a moment where the elements are recaptured for reverse engineering (in the best cases). The maintenance may be made with remote monitoring as well as onsite visits, giving the ability to maintain and even improve performance during use.
In this case, data can potentially be collected during use and after use, providing manufactures with higher levels of real-use data, to be able to improve their products (APSRG, Dec 2014).
An example of Rolls-Royce – ‘Power by the Hour’, is that they retain the ownership of their cores and spares, and charge for the usage. Rolls-Royce takes on the maintenance tasks, and so it is in their interests to maximise performance and reuse capability (Parker, 2007).
Core deposit/Incentivised Return and Reuse: An example, by Parker (2007), is CAT (Caterpillar) who have created a ‘core deposit,’ that is set above the market price for the used part, so that the customer is incentivised to return the used core back to CAT. CAT also offer a full warranty on the remanufactured part, to improve quality assurance.
Schemes can also include ‘buy-back’ systems whereby the OEM/Orginal Retailer (B-to-B, or B-to-C) pays cash or gives credit towards a new purchase.
And USITC (2012), also give the example of some remanufacturers selling remanufactured cores with an additional core deposit price. This deposit is given back to the customer when they return the core (not necessarily the original remanufacturer). This, example also incentivises core return, and a sufficent and relatable source of cores.
Service-Exchange System/Like-for-Like: This strategy, replaces a faulty product with a similar age/aesthetic/condition/model product from the after-sales-service ‘Inventory’; and in examples below, the replacement products have been through ‘Remanufacturing’ or ‘Reconditioning’ reverse-engineering processes.
An example from Sony Playstation Europe (SCE), by Parker (2007) is when a customer contacts SCE regarding a fault, and the telephone team determine that it is a real fault, then they arrange collection and bring the faulty products to a central logistics hub. The faulty product is then checked, and if it shows no signs of abuse, a fully remanufactured replacement is sent back to the customer.
The Sony Playstation system was built on economies of scale and a high level of cannibalisation of reclaimed parts, which brought down warranty repairs to the minimum.
CAT Inc., focuses on packaging for this business model, by providing a customer of a remanufactured part from CAT, a reusable container, for which they pay a deposit. When worn parts are returned to CAT, the customer (should) use the packaging. According to Parker (2007), this has reduced wooden packaging waste by 70% in their Shrewsbury site, and reduced damage of cores during transit.
OEM, Sub-contracted, or Non-OEM (Third Party): This sub-section looks at some of the possibilities of who can actually be doing the ‘Reverse Engineering.’ This is specifically not shown in the map, as all three of these actors could be active on their own, or together, in one revalue chain, or one of them can be mixing strategies across various different product lines (i.e., an OEM is remanufacturing some of its’ products/cores, and sub-contracting the remanufacturing of others).
OEM revalue manufacturer: In this case, the OEM remanufactures its own products at the end of original use. In this case, they have all the information and experience of their products, and the potential to re-design products to make them easier to revalue. They also have access to spare parts, the ability to cannibalise parts for later use, and have access to distribution channels (Sundin, 2004). Below is a list made by Lund 1983 (in text by Sundin 2004), so why OEMs can compete effectively against smaller local remanufacturers:
- “The company had higher worker productivity because of its factory methods;
- it used facilities, specialized equipment, and energy more efficiently;
- the quantities it produced were large enough to justify machines requiring less skilled workers, and
- it salvaged more materials, thereby greatly reducing its requirement for new materials and the cost of new parts.”
Also, by using the existing supply chain network, Jacobsson in 2000 (in text by Sundin 2004), OEMs also have more advantages:
- “Knowledge of the consumer provides the OEM with user patters, which, in turn, are valuable in evaluating the remaining values in the discarded product.
- Detailed information of the consumers and the market for the original product also provide the OEM with advantages in the marketing of the product. First of all, the OEM can estimate the size of the market and remanufacture products according to estimated demand. Secondly, this kind of information provides the OEM with an excellent situation to evaluate the requirements from the customers and which market segments may be interested in the remanufactured products.
- With regard to marketing, the OEM also has the advantage of using its reputation for producing high quality products in the process of convincing the customer of the reliability of the remanufactured products.
- By having the equipment, competence and infrastructure for manufacturing in place, the OEM already has a system that can be reversed. It also reduces the need for investments for the remanufacturing operations.
- The OEM generally produces higher quantities allowing for investments in more advanced production/remanufacturing equipment.
- The OEM is also generally better equipped to earn profit from remanufacturing, as recovered parts can be used in the manufacturing process, providing a higher return than if the parts were to be sold.” (Sundin, 2004)
OEMs can also have a few different ways in which they work within the revalue ecosystem: Full OEM (that does everything – recapture, reverse-engineering, reselling…); OEM with Retailer as redistribution and a ‘Reverse Logistics’ manager; or an OEM working with an independent ‘Reverse Logistics’ company.
Revalue Sub-Contractor: In this case, the OEM usually still owns the products, but outsources the work to a specialist revalue company. The advantage here is that the OEM does not have to invest time and money into revalue activities (and knowledge), and still controls the management of revalue products/cores/components in the revalue chain (RE-marketing), and the Sub-Contractor can specialise – and horizontally specialise, serving other companies, and increasing economies of scale and expertise – and:
“…the company can expect to obtain assistance from the OEM in terms of replacement parts, design and testing specifications, and even tooling.” (Sundin, 2004)
On the other hand, OEMs are careful to manage who they Sub-Contract, due to this potentially high level of IP transfer:
“SCEE [Sony Computer Entertainment Europe] are careful not to work with companies who are also engaged in operations with a direct competitor, this ensures issues of commercial sensitivity are safeguarded.” (Parker, 2007)
Sub-Contractors can also work in different ways: Full Sub-Contractor, which serves 100% the demands of the OEM; Sub-Contractor with independent distribution, whereby they manage the reselling; Sub-Contractor with independent reverse logistics; Sub-Contractor which both manage reselling and reverse logistics.
Revalue Non-OEM (Third Party/Independent): Non-OEM revalue companies often depend on the ‘Core Brokers’ for the sourcing of products/cores (USITC, 2012). This opens up the potential problems with the quantity, quality, and type of core that the core broker can source. This can increase the uncertainties for independent (USITC, 2012).i
Independents also need spare parts, for the remanufactured cores, however, some OEMs actively make it difficult for independent to source spare parts (Sundin, 2013).
Independents also often need of design specifications, to remanufacture cores – and often can not, due to a lack of specifications (APSRG, Dec 2014). OEM’s are (understandably) unwilling to share potential IP with independents, however, the United States has moved some way in reducing this problem, through the implementation of the ‘Freedom of Information Act,’ that allows general access to product information, held by governments and corporate bodies, to individuals (APSRG, March 2014).
And finally, non-OEM revalue companies can suffer from cheap imports of new parts (Sundin, 2013), and the costs of cores, related to scrap prices – when scarp prices are high, core brokers (scrap yards in particular) are more likely to dismantle cars and sell the materials (usually metals) as scrap, than sell the components or parts to other core brokers or remanufacturers (Sundin, 2013).
This final section within ‘Design for Revalue’ is an introduction to further strategies, that can also be applied to the product, which go beyond Recapture or Reverse Engineering efficiency.
Integrative Design: When designing a product or a building, for instance, the ‘whole system’ is identified, by looking at the multitude of relationships between various components, or subsystems, and then designing the entire system for multiple benefits, rather than a single benefit (Capra, Fritjof et al., 2014). The Rocky Mountain Institute (RMI) cofounded by Amory B. Lovins in the United States, has been a key actor in this systemic, nonlinear approach to design of products.
This type of design requires the collaboration of a multi-disciplinary team, such as architects, designers, and engineers; which can bring about huge advances in energy and material efficiency.
‘Integration‘ strategies by RMI include the concept and practice of integrating the four most energy-intensive sectors of the US economy: Electricity, Buildings, Transportation, and Industry. For instance, the problems of energy for automobiles, and the electricity grid can be easier to solve together (rather than separately) (Capra, Fritjof et al., 2014). And extended by Lovins himself:
“Synergies likewise arise from integrating innovations in technology, policy, design, and strategy, not just the first one or two.” (Lovins & EMF, 2013)
This integrative design, at the level of a States’ hard infrastructure, has also been developed by Jeremy Rifkin, with his “The Third Industrial Revolution” strategy – this is developed further in the ‘Hard Infrastructure’ section within ‘Infrastructure and Institutions.’
Ecodesign & Biomimicry: This overlaps with ‘Integrative Design’ mentioned above, as, at its best, it is also systemic in approach, based on ‘loops/cycles’ and non-linear systems.
The Ecodesign work includes the ‘cradle-to-cradle’ work developed notably by Walter Stahel (Product-Life Institute), and McDonough and Braungart (MBDC),’ which is said to be a design approach inspired by nature. These concepts apply basic ecological knowledge to the design of products, buildings, cities, industries and even social institutions (Capra, Fritjof et al., 2014).
Another strategy, within ecodesign in the broad sense (in this map), which has links with cradle-to-cradle design, is the work developed by the Zero Emissions Research and Initiatives (ZERI) organisation, lead by Gunter Pauli. This strategy is focused on recycling waste streams using natural cycles, whereby the output of one activity becomes the input for another. The (potentially new) activity which profits from this potentially low cost input, creates diversity in activities that can create new incomes and jobs. To understand more about how some of the ZERI strategies have been applied, go to the ‘Recycling’ section, and within that, ’Upcycling,’ or here to the ZERI or Blue Economy websites.
Again linked to cradle-to-cradle, and ZERI, is biomimicry. Here, the focus is on learning from nature, which then inspires design of structures, materials and processes. It is a design method, first coined by Janine Benyus in 1997 (cofounder of Biomimicry 3.8), which is also developing in other places like the Biomimicry Institute for example. Benyus’s basic argument is that living organisms and ecological systems have been solving many of the design problems we have, through billions of years of evolution, in efficient and ecologically sustainable ways. In many case this systems design method, brings together scientists from physics, biology and chemistry for example, and puts them together with engineers and designers. Go to the web-links referenced above, to see real world examples of this in action.
“Biomimicry relies on three key principles: Nature as model: study nature’s models and emulate these forms, processes, systems, and strategies to solve human problems. Nature as measure: use an ecological standard to judge the sustainability of our innovations. Nature as mentor: view and value nature not based on what we can extract from the natural world, but what we can learn from it.” (EMF & D&T, 2012)
Eco-design is often a strategy focusing on the life-cycle of a product to reduce its' negative impacts (i.e., emissions such as toxic materials and CO2, and the amount of water, energy and materials used through the value chain). However, this focus on reducing the negative impact when supplying a product, can result in a product that is more expensive. This can be a hard sell to customers - and therefore to people within the companies. Revalue design, however, looks at some of the potential new income streams (as well as cost savings) that are available. Therefore it is less about reducing negative impacts, but more about creating new income opportunities.


Hard Infrastructure: Jeremy Rifkin (2011) reminds us that (hard) ‘infrastructure,’ is at its deepest level, not a static physical range of building blocks (i.e., bridges, airports, sewage systems, and telephone cables); instead ‘infrastructure’ is an organic (and dynamic) – collection of three interdependent (and integrated) core elements/technologies. The first is communication technology, which supports the coordination, and management (including transmission, storage and monitoring) of data and information; the second is the energy technology, which makes conversion and transportation of matter in all our production systems possible; and the third is the transportation systems we use to move all matter (including ourselves) around. Infrastructure, looked at through these three core systems, can give a rich perceptive on the evolution and developments of economies and societies in the past and into the future. It is, in effect, a systemic historic economic view of infracture. Jeremy Rifkin has built on these insights to develop a systemic strategy that focuses on going beyond fossil fuels by bringing together these elements ‘Transport,’ ‘Communications,’ ‘Monitoring Systems,’ and ‘Energy,’ together with Construction (building stock), into an integrated solution. This strategy is known as the ‘Third Industrial Revolution’ (TIR), which, according to Rifkin (2011) contains five pillars: Another systemic strategy in hard infrastructure, which both compliments TIR and extends into other areas (particularly energy efficiency) is ‘Reinventing Fire,’ which has been developed by The Rocky Mountain Institute (RMI) and its’ cofounder Amory B. Lovins (also see the section on ‘Integrated Design & Biomimicry). Like TIR, the ultimate goal is to make the shift from fossil fuels to renewables, using a systemic approach: “…to create a clear and practical vision of a fossil-fuel free future for the United States, backed up by quantitative analysis, and to map a pathway to achieve that future.” (Lovins, 2009) The strategy integrates four of the most energy-intensive sectors in the US economy: Hard Infrastructure: ‘Transportation,’ ‘Energy,’ (electricity in particular), with ‘Industry,’ and ‘Buildings’ (Construction). For ‘Transportation,’ the three main innovations are based around making vehicles ultra lightweight; hyper aerodynamic; and powered by hybrid-electric drive. For the ‘Energy’ used for ‘Transportation,’ ‘Industry’ and ‘Buildings,’ the three main innovations are radical energy efficiency (retrofitting commercial buildings, or capturing and reusing waste heat for example); switching to renewable sources of electricity such as Wind and Solar power, hydrogen fuel cells and advanced biofuels for example; and the integration (like Rifkin) of the ‘Communications,’ ‘Monitoring,’ and ‘Energy’ (the Electricity Grid in particular) known as the “smart grid” (similar to Rifkin’s ‘Intergrid’), which: “…is an electric system that uses a multitude of smart chips and instant communication to interlink and coordinate countless small generators whose lower costs, lead times, and financial risks make the system far superior to a centralised grid.” (Capra, Fritjof et al., 2014) And in particular, this section focuses specifically on energy or biomass recovery from biological waste (or sometimes a secondary output), not those crops – such as sugar, palm-oil, and corn, that are specifically grown for biofuel production. Anaerobic Digestion: Energy can be extracted from biological materials in various processes, with incineration and anaerobic digestion (AD) being the most typical. The energy that can be recovered from AD can be in the form of heat, cold (with the use of a heat exchanger), and a gas that can be burnt to create electricity, or further processed (through transesterification) to create a liquid fuel – biodiesel, or separated into pure carbon and hydrogen (Pauli, 2015). A further output is the semi-digested slurry, which can be used as a form of fertiliser. Anaerobic Digesters, can be built in varying scale, from those, typically in India, that are used at the household scale, to large industrial scale digesters with a capacity in excess of 10,000m3 in China. The output quality and quantity, is directly linked to the inputs used. A vast amount of organic materials can be used in AD, however the type and amount of different elements within the feedstock (i.e., hydrogen, carbon, oxygen and trace elements) has a huge impact on the output yields of gas, and the quality of the semi-digested slurry. Many AD plants are not running at full capacity, as the mix of inputs are not correct (The Organic Stream, Dec 2015). One of the key advantages of AD is that, in the theory of cascading, it uses waste as an input to bring multiple benefits. However, particularly in Italy, many AD plants have been built by investment groups, and with an eye on yield, have in many cases, opted for the use of corn as the main feedstock (to increase stability in sourcing the feedstock). In this case, this primary product, corn, has been grown for the use within the digester, and therefore, is not part of the cascading waste cycle, and places AD squarely in the same controversial issues with biofuels – plants grown for fuel (i.e., sugar, corn, palm-oil), and their use of energy to grow and competition for food (and therefore price) and land… AD is very popular in Germany, with over 10,000 AD sites currently in operation nation-wide, and is a great example of de-centralised local energy production (i.e., local management, investments and profits, and local inputs and outputs). However, most of those sites (according to Pauli, 2015), are mainly existing through subsides, and as mentioned above, if using non-waste feedstock, are far from carbon neutral. Syngas: Although ‘carbon capture,’ and capture of other green house gases (GHG), from the polluting smoke stacks of industry, has seemed a dream technical ‘patch,’ it seems that a company in New Zealand (LanzaTech), has found a way to convert syngas (CO2, CO, and H) into ethanol. By using specific microorganisms to ferment the syngas, in a proof of concept project, 100,000 gallons of ethanol has already been captured from the chimneys of a steel mill in China (Pauli, 2015). Aerobic Digestion (Composting): Constantly rebuilding healthy soil, is one of the foundations of sustainable agriculture, and sustainability of the natural environment in general. One way to build new soil, to support this thin layer that covers the Earth’s landmass, which has taken ten of thousands of years to develop, can be through adding compost and humus. Much of the different actors in sustainable agriculture look beyond the synthesised NPK (nitrogen, phosphorus and potassium), and look more to the compost as a medium comprising of the right soil-biology, with the right mix of materials for feeding that biology when it is added to the soil. Compost can be produced through various processes, ‘cold,’ ‘hot’ or vermi-compost (with worms) are typical examples. These processes are possible at the level of an individual farm or ecological cluster of farms; and if done well, can both increase (Upcycle) and improve (in quality and in time) the availability of nutrients to the soil – via microorganisms in the soil – for plants; reduce the amount of (or at least, the speed in which) elements are ‘leached’ into the atmosphere, particularly those which are also GHGs, and those leached into the soil (and therefore groundwater) and sea; and reduce the need for external and synthetic fertiliser (see ‘Agroecology’ for more examples). The following elements: ‘Electric Plug-in & Fuel-Cell Vehicles,’ ‘Internet-of-Everything,’ (which including communications and monitoring systems), ‘Renewable Energy, Storage and Intergrid,’ are part of some of the technical innovations within the framework of industry 4.0. Go here to find out more. Fresh (no salt and clean) water may prove to be our most limited resource in the future. Keyline design(R) developed by P. A. Yeomans for capturing water on landscapes, the work of groups harvesting water in cities, green-roofes, regenerative soil farming practices, and ecology (i.e., replanting trees, bringing beavers back) are just a few of the strategies around the world, that are being to used to improve water capture on landscapes.
The following section highlights some insights on ‘Recovery‘ within the ‘Recovery, Renewable Energy & Storage, & Integrid.
Industry 4.0
Water Systems (fresh and waste)
Green Architecture: This is used as an umbrella term in this map for all the ecodesign strategies used in construction and retrofitting (the addition of new technologies or features to older systems) of buildings.
Green architecture can use ‘bio-mimicry’ (see ‘Integrated Design & Biomimicry’ for more information), or principles from nature as inspiration, in designing the structural form, to take the greatest advantage of the Sun and the Wind, for maximising solar heating, and cooling, ventilation, and natural light. The termite mound, for example, has been used as one of natures ‘teachers’: Termites (in Zimbabwe) are able to build huge mounds, where them farm fungi for food. The fungi grow best at around 30oC (87 oF), however, the temperature outside vary from 35 to 40oC. And so, termites have evolved a system of constantly opening and closing a series of heating and cooling vents, within the mound, throughout the day. By carefully controlling the convection currents, fresh air is drawn into the lower part of the mound, and then channeled through to the peak. As the environment is dynamic (not static), the termites are constantly digging new vents and filling old ones, to maintain the temperature over the seasons or due to other local environmental changes (inspired by article retrieved on the 13/12/2015 from inhabitat.com)
Systemic architect Anders Nyquist from EcoCycleDesign, has designed and built, houses and schools based on the ‘termite technology.’
Green architecture also involves highly efficient forms of insulation for all construction surfaces including windows, that in some cases can make space heating systems redundant. Buildings, can also be designed to capture and distribute water from the rain into the house for certain uses, or outside the house for irrigation systems for the garden for example.
Finally, like the strategies mentioned above in ‘Hard Infrastructure’ by Rifkin and Lovins, a building can become not only an energy saver, but also an energy producer, with the use of different forms of solar panels and solar water heaters, or even biogas for example.
Regenerative agriculture is often used as an umbrella term for many (if not all) the systemic, and soil and diversity building agricultural strategies.
Agroecology: Modern agriculture often promotes the use of agrochemicals and monoculture design, which weakens ecosystem resilience, reduces biodiversity, and undermines Earth’s ability to provide ecosystem services. Modern methods can also have a negative impact on public health, the quality of food and its nutrition. Socially, it impacts traditional rural livelihoods and cultures; and psychologically can separate farmers from the land as an ecosystem. Economically, it also creates a debt cycle for farmers, and is often focused on exportation, and framing food as a commodity (no qualitative differentiation across a market).
Agroecology on the other hand, aims to build on the traditions/cultures and skills of the past and studies many developing countries (where they have low means, and therefore low external inputs), and mixes this with modern scientific understanding of ecology, ethnology and sociology for example. The result is a focus on developing agricultural systems specific to the location (as all locations are unique), and balancing outputs with the continuous building of soil fertility, natural pest control, low external inputs (except knowledge), potentially more jobs, and helping to put nature back in line with the path of evolution.
As Gleissman (1998) underlines, that agroecology, from a management perspective, is focused on providing a balanced environment, biologically mediated soil fertility (soil-food-web) and pest regulation, and sustained yields, through the diversified various methods and low-technology inputs. One of the key principles of agroecology is diversification of farming activities through biodiversity, and mimicking nature. Some strategies include intercropping, agroforestry, and other diversification methods, that are able to mimic natural systems, and therefore greater resilience within changing (as a greater diversity of species has a greater chance of surviving – over all – not all species).
Below is a list of agroecology strategies:
- Crop Rotations: In soil where the soil-ecology (life) is not in a healthy state, then pests and weeds can take hold in repeating annual crop systems. One answer is to rotate crops into different places (and vary the yearly sequences), so that weed and pest cycles can be reduced or stopped. Another reason, is that certain plants supposedly ‘use-up’ more of one nutrient than another, and so by moving the plants around, then the land can ‘rest’ in-between seasons, so plants don’t strain the system. However, others believe that all plants needs more-or-less the same nutrients, and if the soil-ecology is healthy (and by definition, the soil is aerobic), and a diverse system of cover crops is established, no rotation is required: IF the soil-ecology fits the plant type, then, in principle, no weeds or pests will be able to dominate. Rotations can be made within similar plant types (which is a bit counter-intiituive for the traditional rotation theory).
- Polycultures: Complex cropping systems can place different plants that grow at different times, or in different spaces (i.e., high or low) for instance, to maximise photosynthetic production of land space (in 3 dimensions); and to increase diversity, which creates habit for beneficial predators, traps for pests, protection zones from the elements for instance – and diversity means greater potential of resilience over time, as change in systems is inevitable, and so diversity increases the chance of survival of at least one species during times of change (i.e., hot or dry periods).
- Agroforestry Systems: Linked to polycultures, this sets trees apart, as trees are an incredibly important plant, that has multiple benefits for landscapes, such as increasing rainfall, and reducing adverse weather conditions, holding rivers and land on slopes together, drawing up deeper waters and nutrients, and high densities of diverse species life on trees; to name a few. Trees are also high yield in food, fibres, and chemicals.
- Cover Crops: Again, mentioned in rotation, and can also be a polyculture; however the key functions of cover-crops is to keep the land covered – reducing evaporation, and increasing nutrient cycling all year around; and again, creating habit for beneficial predators. And cover-crops can also bring in nutrients, such as nitrogen-fixers, or can be a secondary crop for hay or directly for animal pasture.
- Animals: Integrating animals – particularly herbivores (and even their predators) – back into our farms and landscapes, is seen as a key way to regenerate natural grass-land habitats (where most of our crops are grown). In these environments, herbivores would have co-evolved with these plants, and so the landscapes can benefit from well-managed herbivore-plant systems – such as in Holistic Management, to improve particularly ‘brittle landscapes.’
As a final note, some of the work of the Blue Economy, has been the development of business models that attempt to make some of these strategies both economically viable and job positive (creating new jobs). See their case studies on the website link given above for more information.
Urban Agriculture: Within this map, ‘Urban Agriculture’ is used as an umbrella term for three main activities: Edible Landscaping, Community Gardening, and Urban Farming.
Urban Agriculture is a growing global movement (particularly during the last 10-15 years), which is also recently being integrated into agroecology (see Altieri’s work in urban agriculture here for example), and permaculture (Toby Hemenway for example). As society is becoming more urban, it seems that these strategies (i.e., agroecology and permaculture) are also moving logically to where people are…
Urban agriculture is defined as the practice of agricultural production, processing, and distribution in, or close to, towns and cities (Bailkey, 2000). And to be clear what is meant by ‘Urban,’ is the regulatory boundary of a municipality and not to do with density. And so some very spread (low density) urban environments may not seem very ‘urban’ compared to others (EcoDesign Resource Society, 2013).
And below are definitions of the three forms of Urban Agriculture:
- Edible Landscaping: These are typically cubically owned/managed, and designed with edible fruits/berries/nuts for public consumption. These landscapes, are in general maintained by the city or volunteers. (Creasy, 2009).
- Community Garden: These are typically the gardening or growing of food, by groups, individuals or families, in a shared garden space. These gardens are often on public land. (American Community Gardening Association).
- Urban farming: This is the growing, processing and distribution of food within the city limits for a revenue. Other names for this type of farming is, market gardening, commercial agriculture (EcoDesign Resource Society, 2013).
And finally a last word from George Orwell from his book the ‘Road to Wigan Pier’ (1937):
“I think it could be plausibly argued that changes of diet are more important than changes of dynasty or even of religion….Yet it is curious how seldom the all-importance of food is recognized. You see statues everywhere to politicians, poets, bishops, but none to cooks or bacon-curers or market gardeners.”
This section focuses on those institutions that make up the public and private ‘Soft’ infrastructure of a nation state. ‘Soft’ is distinguished from ‘Hard’ (explained in the next section), not by physical structures – Soft Infrastructures often have them (i.e., hospitals, schools and government buildings), but the focus is on the economic, health and cultural and social level of the state; whereas ‘hard’ infrastructure is more about the means (bridges, airports, fibre-optics, satellites, trucks…) in which elements (people, goods, information, energy, water, waste…) flow around the economy.
In the section ‘Hard Infrastructure’ the focus is on some of the broad strategies in systemic sustainability (particularly in transitioning to renewable energy). However, in this section, the ideas discussed are focused on those that have direct relevance to the ‘Revalue Chain.’ So, for example ‘Health’ is not discussed here (but if you want to know more about some interesting strategies in health see the work of John Thackara here, or Lester Brown and the Earth Policy Institute here); and neither is ‘Social Welfare’ (though of course, they are both important elements). There are also a number of ‘soft-infrastructure’ elements that are not shown on the map, such as ‘Media,’ ‘National Security, Emergency Services and Military,’ ‘Judiciary System,’ and any of the groups that are part of ‘Civil Society’ like ‘Activist Groups’ or ‘Volunteer Groups’ for example.
Tier 1 (The 1st column from the left in the map)
State or Region: A state or region can have a revalue strategy (and many do), however, this will be looked at in the ‘Development Policy,’ which is within ‘Tier 3 & Flows.’
Governance, Economic, Social, and Cultural and Civil Society institutions: In this map, ‘Soft Infrastructure’ has been broken down into these four main groups.
Tier 2 (The 2nd column from the left in the map)
Legislation System: Legislation is regarded as one of the three main functions of government (‘Executive’ being the second – which is also shown in the map, and ‘Judiciary’ being the third – which is not). Legislation (aka ‘statutory law) is law that has been put in place (enacted) by legislation or other governing body. Legislation is used for various purposes, from regulating, authorising, proscribing, to provide funds, sanctioning, or to declare or restrict for instance.
Below are some examples of different types of UK, and European legislations that have an effect on different revalue activities:
Product Take-Back Laws: Sweden has put in place laws, which oblige manufacturers to take-back products at the end-of-use. This has encouraged manufacturers to find and develop solutions for recycling and product life extension, to recapture value out of these products and materials, that otherwise would only be a cost. (Sundin, 2004)
Landfill Directive: Landfill taxes, are generally increasing – due to reductions in capacity, and reductions in regions wanting to support this form of waste management. Rises in landfill taxes is provoking the exploration for alternatives, such as product life extension and recycling, which can not only reduce taxes, but generate incomes. (Gray et al, 2007)
Waste Electrical and Electronic Equipment (WEEE) Directive: The WEEE Directive aims to reduce landfill, by providing the impetus to boost recycling. WEEE states that all buildings and shops used to store used products have to be registered to handle and store ‘hazardous waste.’ All products (since April 1st 2007) have to be marked with the WEEE symbol in the UK for instance. (Gray et al, 2007)
Waste: The fact that end-of-use products are classified as waste at the end of their first use (if going through waste collection channels), creates barriers to the revalue chain. This classification restricts the type and number of goods or components that could easily go through life extension processes; mainly as it makes it more expensive, as the protocols for handling waste are much more strict (and therefore expensive), than the actual context of revaluing used products (in most cases) requires; or in more extreme cases, it actually makes it illegal to collect these used goods, which are classified as ‘waste,’ revalue them, and resell them on the market.
RoHS: This European Directive states that if part of a product is replaced, the entire product has to be reassessed for the CE (Conformité Européene). This has created a legal ‘black hole’ for life extension activities, such as remanufacturing (APSRG, 2014).
Freedom of Information Act (FoIA): The FoIA attempts to provoke the right to access of information, which is held by governments and private corporations. In the USA, this has allowed independent remanufactures access to OEMs’ product design specifications, so that they are able to remanufacture products to the same quality standards (Gray et al, 2007).
Sale of Good Act (SoGA): The SoGA puts the burden of faulty products on the retailer, and therefore, not on the manufacturer; and so, does not encourage manufacturers to make any revalue adaptions to their products (APSRG, Dec 2014).
Trade Description Act (TDA): “The TDA prevents manufacturers, service industry providers and retailers from misleading consumers as to what they are purchasing. Remanufactured products are often considered by consumers to be less reliable than new products. Following the recommendations surrounding a legal definition of remanufacturing and certified mark for remanufactured products could increase consumer acceptance.” (APSRG, Dec 2014)
The EU Waste Shipment Regulation: “The EU Waste Shipment Regulation bans all exports of hazardous waste to non-OECD countries and all exports of waste for disposal outside the EU. Although this is a very important and extremely necessary piece of regulation, industry members interviewed during our inquiry stressed the need for both remanufactured items and items due to be remanufactured to not get caught up in this regulation.” (APSRG, Dec 2014)
The Energy using Products (EuP) Directive: “Remanufactured components may not be as energy efficient as new components of more recent design. The EuP Directive is continually revised to reduce standby and in-use energy consumptions. There is the possibility through this Directive that it could become impossible to sell remanufactured products if they use more energy than new, low-energy models. Although lowering stand-by and in-use energy consumption is beneficial in isolation, it may not be the best measure to drive holistic improvement. A more holistic approach focusing on the entire supply chain of products should be used to assess energy-saving potential. This Directive is particularly relevant for large electrical appliances such as white goods, which have a high potential to be successfully remanufactured.” (APSRG, Dec 2014)
Recycling Targets: “Although the EU is recognising the potential of the remanufacturing industry by funding numerous research and development projects in this area, legislation is still largely focussed around recycling rates with the most recent EU recycling targets set at 50% by 2020 and 70% by 2030. Some companies, for example Lexmark, have begun to set internal management targets that aim to reduce recycling rates, enabling them to achieve higher rates of remanufacture. European policy makers have perhaps not recognised that to move on to the next stage of a resource efficient economy the familiar policy framework favouring recycling may need to be reversed. By continuing to promote materials recycling, the policy framework may hinder the development of product remanufacture in Europe.” (APSRG, Dec 2014)
Government Procurement: Public spending can act as a ‘lead-user’ to help drive consumption changes in regions. For example, in the U.S. there have been policies to move procurement of performance-based services (rather than goods), which has created a large scale of demand. Governments can also play the role of ‘matchmaker,’ helping to bring different actors of the value chain together to drive for new opportunities (EMF, 2013).
Executive System: Is the government party that exercises authority in and holds responsibility for the governance of the state or region.
Tax System: Tax is a financial charge (or other form of levy) imposed upon a taxpayer by the state to fund various public expenditures (i.e., pay for the construction and maintenance of public infrastructures and institutions).
Taxes can also be used to transfer wealth (particularly through ‘Social Welfare’), and Taxes can also act like ‘Legislations,’ which can be used as economic motivators to regulate, increase, or to restrict certain forms of economic activity. Here Stahel & EMF (2013) outline how tax systems could be shifted to promote revalue activities (and other activities that can also help reduce the impact of linear economic activities):
Tax Shift: “…adapting the tax system to the principles of sustainability by not taxing renewable resources, including work.” (Stahel & EMF, 2013)
Not taxing work – human labour, and not charging VAT on revalue activities, could encourage the activities that have a positive effect, whilst discouraging those that don’t. The resulting loss of income to the state may be able to be compensated by taxing the consumption of non-renewable resources (i.e., materials, energy, wastes and emissions) (Stahel & EMF, 2013).
VAT: In the US, enterprises are able to benefit from an accelerated deprivation of 50% of the adjusted basis of assets purchased for product life extension or recycling of waste materials/goods. Furthermore, an enterprise that purchases waste materials/goods and reuses them for manufacturing processes, is able to recover a deemed input VAT. In China, an enterprises’ revenue from ‘synergistic use of resources’ can be reduced to 90% of actual, when calculating the taxable income; and goods made from recycled materials (since 2011) have limited or zero VAT, which encourages revalue activities (EEF, July 2014).
And some suggestions from the RREUSE network, around VAT are as follows:
- Zero VAT on product life extension work, and sales;
- The allowance of retailers to recoup VAT through donations of unsold products to accredited/approved resuse centres from the social economy;
- Zero VAT for product life extension activities carried out by social enterprises (RREUSE, Sept 2015)
Here, are few additional Tax innovations, which go beyond direct revalue activity promotion:
CO2 Tax: A price on CO2 emission in developed countries. This could raise (at $50/ton) an estimated $450 billion annually, according to the World Bank, the IMF, and the Organisation for Economic Co-operation and Development (OECD) (Klein, 2014).
Free Trade for Organic Produce: A free trade (no import – or export – taxes) on organic produce. It would require an agreed definition of what is classed as organic (Pauli, 1998).
Financial System: Is the overall system that includes financial regulation, the printing and management of the national currency, national accounts and the management of the national budget, and more recently local currencies, and digital currencies are also entering into this area.
Manufacturing & AFFF Infrastructure: This includes the soft infrastructure around manufacturing such as ‘Parks and Zones’ for Manufacturing which is discussed later, and it overlaps with ‘Hard Infrastructure’ and ‘Legislation’ specific for Industry. This also includes soft infrastructure for AFFF (Agriculture, Forestry, Fisheries and to some extent Foraging), which can include agricultural price support, insurance, quota management and related enforcement systems, and regional storage facilities for example.
This group also includes programs put in place which provide access to the latest manufacturing technology and expertise for Manufactures. An example of this is the Catapult: High Value Manufacturing program in the UK, which is similar in some ways to the Fraunhofer (a group of nearly 70 applied scientific research centres around Germany).
The program has grouped together 7 ‘world-class’ manufacturing centres, with the goal to provide access to for companies to test the latest manufacturing technologies, supporting them in prototyping and testing pre-industrial runs prior to investment in the technology in their company, and providing access to knowledge/expertise to both help the companies develop their solution, and if required, train workers in the final application.
This type of innovation could help SMEs in particular, where capital investment can be a huge barrier to entering certain revalue activities, particularly those manufactures wanting to move towards remanufacturing where new specialised capital good technologies will be required.
This example has overlaps with ‘R&D Labs,’ but is placed here, as the labs and manufacturing facilities themselves do not (yet) have specialised knowledge and skills in revalue activities (as the R&D labs do), it is more an innovation in how these facilities are grouped together and made available to industry. This group is also linked to ‘Fab-Labs.’
Education System: This is the overall education system from primary, secondary, further education (FE) and higher education (HE). The Ellen MacArthur Foundation (EMF) is an example of a group that is working at many of these different education stages to help educate people in the concepts and potential of the circular economy. See here for more information.
Tier 3 (The 3rd column from the left in the map)
Principles/Standards/Guidelines: Below are some of the main standards that are already used in industry that can be used as a beginning platform for revalue activities.
ISO 14001: There are currently two main regulations/standards on the market. The first is EMAS (see below), and the second is ISO 14001. ISO 14001 is a global certification, where external auditors from accredited firms perform audits to make sure companies fulfil standards to hold the certification. (Sundin, 2004)
EMAS: The second main regulation/standard (after ISO 4001 see above), is EMAS – Eco-Management and Audit Scheme. EMAS is a European certification (Sundin, 2004).
Development Policy: These are the policies that governments make that activity aim to promote a particular activity/set of activities over another. Governments can do a lot in helping change the ecosystem in which industry is present, to encourage and favour revalue activities over the traditional linear economy (extract, make, use, waste).
China, Japan and Germany, have shown that when a coherent future vision has been framed, and objectives aligned, a driving force can be developed for change at the level of the system (EEF, July 2014). Below are some examples from different countries (based around remanufacturing):
Japan: This country has a relatively well-developed remanufacturing industry, and design for revalue expertise in durable goods, such as photocopiers and single-use cameras, but with low activity in the more typical sectors, such as the automotive industry (ASPRG, Dec 2014).
China: China has made a strong focus on supporting remanufacturing through regulation. Other strategies include cross-departmental pilot programmes for motor vehicle parts, industrial machine (capital goods), and electrical equipment sectors; and a strategic road for implementation (APSRG, Dec 2014).
Singapore: The Singapore government, in 2011, launched the Advanced Remanufacturing and Technology Center (ARTC), an R&D center that works with local universities and remanufacturers to develop remanufacturing technologies for aerospace, motor vehicle parts, marine, and HDOR equipment sectors. The center has partnered with a handful of SMEs and larger multinational companies, including Boeing (U.S. ownership), Rolls-Royce (UK ownership), and Siemens (German ownership), among others (UTISC, 2012).
Scotland: The Scottish government in 2014 announced a 1.3 million pound sterling funding of the Scottish Institute of remanufacture. Since this time Zero Waste Scotland and other Scottish (and British) organisations have collaborated to make Scotland one of the most dynamic example regions in the world for revalue activities.
And here are some broader development strategies focusing on the transition to renewable energy:
Feed-in Tariffs: Under a feed-in tariff, eligible renewable electricity generators, such as homeowners, business owners, and private investors, are paid a cost-based price for the electricity supplied to the grid. In most cases the majority of the renewable electricity is produced by large plants, however, financial systems, such as government backed loans to SMEs (Small to Medium Enterprises) and homeowners could help pay for more small scale, distributed (decentralised) power generation projects (Rifkin, 2011).
Phasing out Fossil Fuel Subsidies: According to a 2012 estimate, by the Oil Change International and the Natural Resources Defence Council in the US, phasing our fossil fuels subsidies, globally, could save governments (conservatively) around $775 billion per year (Klein, 2014).
Parks & Zones: This includes two, sometimes linked, industrial development strategies. The first are industrial parks (IE) (also known as industrial estates or trading estates), which are zones that have been planned for the development of industry. The second are special economic zones (SEZ), which also includes (FTZ), EPZ (Export Processing Zones), FZ/FEZ or BLP for example.
Parks & Zones are often created to encourage the entrance of foreign firms into an region, however, this is not the objective here. Parks & Zones can potentially be used as a way to, or at least prototype the, implementation of some of the innovative tax, legislation and development policies discussed above in a smaller ‘controlled’ zone, and at the same time allowing those companies inside to ‘circumnavigate’ some of the issues with existing tax, legislation and development strategies. Zoning, similar to subsidies, should probably be seen as a transition to zero i.e., at a certain point the zone is disbanded, as the outer zone catches up. An example from China, is the Circular Promotion Law, which signals a commitment to embed these strategies into industrial structures. The Law requires plans on the distribution of different sectors of the economy, and regions, by using industrial parks and zones, to help encourage cooperation between different sectors of the revalue chain. Enterprises in these areas, are encouraged to exchange wastes, energy, land, and water, and share infrastructure facilities; which are often combined with Tax incentive strategies (EEF (July 2014).
And another example from China described by USITC (2012), is the motor vehicle sector, where imports of certain goods, such as cores, are aloud into export processing zones (EPZs), for subsequent remanufacturing and exportation. However, China does not allow the import of these same cores into other territories of China, so not to compete with local remanufactures selling cores within China.
Financial Institutions: This includes different types of institutions that deal with Deposits (Banks, Building societies, Trusts…), Contracts (Insurance and pensions), and Investments (Investment banks, Underwriters…). These types of Institutions can be encouraged by government to invest in revalue projects, and if possible, develop credit schemes that are inline with revalue activities.
Revalue Investment: The UK Green Investment Bank is a funding institution created in 2012 by the UK government, with the objective to attract private funds for financing the private sector in environmental preservation activities (therefore, including revalue activities). However, the Bank has come under much criticism since it’s creation, and so in principle the initial concept seemed to have great potential, but the reality is still far from a clear benchmark.
The following example strategies in Finance, are more general, and go beyond revalue activities:
Disinvestment: Modelled after the campaign in South Africa to stop investment in the Apartheid regime, the latest campaign of 350.org is a movement to reduce or even stop investment (stocks, bonds or other investment funds) in fossil-fuel companies, in order to overcome resistance to responsible climate policies (Capra, Fritjof et al., 2014). This is currently mainly targeted at corporations and/or universities.
Green Mortgages: Here, banks and other lending companies, could provide low interest loans to businesses and homeowners, for the installation of solar panels. Assuming that the payback on energy savings is around 8-9 years, homeowners, for instance, with a twenty-year mortgage would be saving money on electricity for 11-12 years in within the mortgage period. The monthly savings on electricity bills, could be leveraged against the mortgage repayments, and could be the basis for a reduced interest rate (Rifkin, 2011).
Green Investment: A study by the Canadian Centre for Policy Alternatives compared a public investment in a $5 billion pipeline (such as the Enbridge Northern Gateway), and a $5 billion investment in a range of green growth activities, including public transit, building retrofit, and renewable energy. The study found that the pipeline project would create short-term construction jobs, and centralise profits in a few sectors of the economy (whilst producing environmental damage and future costs); whilst the green growth activities could create at least three times as many jobs in the short-term, and no to low future costs (Klein, 2014).
R&D Labs: This includes specialised institutions, which can (and often are) linked to a university; but are very much linked to applied studies, research and knowledge development in revalue activities in industry. One of the worlds leading revalue R&D Labs’ is the The Center for Remanufacturing and Resource Recovery (C3R®) at Rochester Institute of Technology in the (US), which since 1991 has worked to develop, text and implement remanufacturing processes that are efficient and cost-effective; whilst promoting the design of products to further increase the remanufacturing potential at the end of use.
And in Germany is the new remanufacturing centre, linked with the Fraunhofer at Bayreuth University, who’s experience goes back many decades.
More recently is a the Advanced Remanufacturing and Technology Centre (ATRC) in Singapore, which is comprised of Manufacturers (i.e., IHI, Siemens, Rolls Royce, 3M, ABB, Carl Zeiss, and TRUMPF) and Nanyang Technological University.
Scotland has also made a continued commitment recently to revalue activities with the creation of the ‘Scottish Institute for Remanufacture,’ which s funded by the Scottish Funding Council and Zero Waste Scotland; and is hosted at, and built on the experience from, the University of Strathclyde. with these three objectives:
- Increase innovation
- Increase activity and engagement from the academic community to build capacity.
- Establish the Scottish remanufacturing community.
And there is also GSCOP in France.
Any region wanting to develop revalue activities, should consider developing a collective centre of development and knowledge, to both reduce the local costs to new knowledge, and increase the local specialised but broad expertise required.
Higher Education: As mentioned above, this links to the ‘R&D Labs,’ however not every University involved in revalue activities has a specialised R&D Lab. Examples include:
University of Strathclyde (Scotland), WASEDA University (JP), Nanyang Technological University (Singapore), Fraunhofer IPA & University of Bayreuth (DE), TUBerlin (DE), and University of Cambridge Institute (UK).
Knowledge Networks: This group also overlaps with R&D Labs and Higher Education, but again, there are independent groups helping to build and spread knowledge in revalue activities that are neither an R&D Lab or a Higher Education Facility. Here are some below:
Ellen MacArthur Foundation (UK), ERN, Duxes Reman Industry Focus, The Knowledge Transfer Network (KTN) and _connect (UK), High Speed Sustainable Manufacturing Institute, , WRAP (UK), APRA.
As with ‘R&D Labs,’ regions wanting to develop their own revalue strategy should be thinking of how to build on existing expertise a specific local knowledge network on revalue activities.
Action Groups: Action Groups can be a mix of any of the actors across the revalue chain and Infrastructure and Institutions. These groups are those that have been formed, to promote and help build strategies and tangible projects in revalue. Here are a few examples:
Zero-Waste Scotland, The All-Party Parliamentary Sustainable Resource Group UK, Innovate UK: Technology Strategy Board, Scottish Institute for Remanufacture, and H-Enea Living Lab (SP).
And like ‘R&D Labs’ and ‘Knowledge Networks,’ ‘Action Groups’ should also clearly be created locally, and as revalue is systemic, these working groups (or clusters) should be promoted as a common platform that brings together many of the actors in the revalue chain together, looking to build collective benefit. This common platform requires a mix of technical knowledge, and also experience of building and maintaining trust and dialogue.
Flows: These flows (Financial Capital, Technology, Information & Expertise, (skilled) Workforce, and Raw Materials – including in this case cores) are the main flows through the economy. All these inputs have corresponding outputs (Profit, Efficiency, IP, Jobs, Goods and Services) once they have passed through the value chain.
Many of the barriers to revalue activities (and, in fact any industrial activity) are often based around, to some extent, the impedance (‘friction’ in economics) to the development or maintenance of these key flows, which all need to be present at a minimum level (Flows do not include other potential barriers, such as the importance of power in the value chain, which is linked to access to markets, and the different forms of competition for instance).
Many of these activities are systemic - that is to say, they involve multiple stake-holders, across industrial sectors and public sectors, and require diverse (and new) skills sets - and so the system 'below' (the infrastructure) needs to support these new collaborations, and relationships, to also increase their existence and potential. As with 'RE-DESIGN,' this can focus on causes, and effects, barriers and opportunities, and start-of-pipes, and end-of-pipes... Public departments, education, research, investors and ecologists play a key role in this section.

SHORT ARTICLES ON REVALUE
If you want to find out more about Revalue, select any of the blog posts listed below.
[wp_blog_designer id="8"]